Rice is one of the most frequently consumed foods worldwide. A wide range of pesticides have been utilized to produce rice to control diseases, pests, and weeds to boost crop yield. Pesticides used in rice crops are typically country/region-specific because of the differences in legislation, weather, and production system.
Pesticide residue in rice influences the quality of the rice but also jeopardizes the health of general consumers. To avoid unnecessary health risks, it is crucial to track and regulate the presence and levels of pesticides in rice.
Many countries such as the United States, China, India, Japan, Brazil, and the European Union (EU) have established maximum residue levels (MRLs) of pesticides for food and feed including rice.1-3
The EU MRLs for pesticide residues in rice generally range from 10 µg/kg to 8000 µg/kg contingent on the pesticide.1 To identify low levels of pesticides in rice demands highly sensitive, selective and accurate analytical methods.
As there is the potential that a large number of pesticides are used in rice production, using multi-residue methods with the capacity to determine many pesticides in a single run offers the most efficient approach.
Generally, pesticide residues were analyzed primarily by gas chromatography/mass spectrometry (GC/MS) methods,4, 5 but GC is not an appropriate technique for ionic and polar compounds, particularly for compounds that are thermally unstable in the GC injection port. Liquid chromatography tandem mass spectrometry
(LC/MS/MS) is now the preferred method for pesticide analysis because of its high selectivity and sensitivity as well as its suitability for a diverse range of compounds in different sample matrices.6-10
QuEChERS extraction method has been applied extensively for the analysis of multi-residue analytes in food samples including rice.4,8,9,10 During this study, a fast, sensitive and selective multi-residue method was developed for the analysis of more than 200 pesticides in rice samples by pairing a modified QuEChERS extraction method with LC/MS/MS.
Utilizing time-managed MRM™ in the QSight® triple quadrupole mass spectrometer, the ideal dwell time of numerous MRM transitions can be produced automatically for the targeted analytes.
This conserves time in the development of the method and enhances data quality and analytical performance, as shown in this study by the results of multi-residue pesticide analysis in rice samples.
Experimental
Hardware/Software
Chromatographic separation of pesticides was performed using a PerkinElmer UHPLC System and determination of analytes was accomplished utilizing a PerkinElmer QSight 220 triple quadrupole mass detector with a dual ionization source. All instrument control, data acquisition, and data processing was conducted using Simplicity 3Q™ software.
Method
Sample Preparation Pesticide standards were acquired from ULTRA® Scientific (North Kingstown, RI). Rice samples were obtained from local grocery stores in Ontario, Canada. Various rice samples such as brown rice, black rice, and white rice (including Jasmine, Basmati and Calrose) were tested in addition to two brands of organic rice samples.
The countries of origin of these rice samples included Thailand, Vietnam, India, Italy and the U.S.
Preparation of the rice samples was conducted in accordance with a published procedure specifying minor modifications using QuEChERS kits (AOAC 2007.01 method) without dispersive SPE clean-up.10 One (1) µL of the extract was directly injected onto the QSight LC/MS/MS system for assessment.
For the controlled blank matrix, an organic brown rice sample was used. Recoveries from the rice sample matrix were assessed by fortifications of pesticides at concentrations of 10 and 100 µg/kg. Calibration curves were constructed by preparing eight levels of standards in a neat solution (acetonitrile) and in the rice sample matrix (matrix-matched calibration).
Matrix effects were assessed by contrasting the slopes of calibration curves acquired from the neat solution and rice sample matrix.
To limit the number of false positives and negatives, a minimum of two MRM transitions were observed for each pesticide. LOQs (limits of quantification) were quantified predicated on a minimum S/N of 10 for both transitions.12
LC Method and MS Source Conditions
The LC method and MS source parameters are displayed in Table 1. An incomplete list of the multiple reaction monitoring mode (MRM) transitions of the pesticides studied is illustrated in Table 2.
The acquisition MS method is automatically generated by choosing the pesticides of interest from the compound library integrated in the time-managed-MRM module of the Simplicity software, this includes both positive and negative analytes.
Table 1. LC Method and MS Source Conditions. Source: PerkinElmer Food Safety and Quality
LC Conditions |
LC Column |
Brownlee, SPP Phenyl-Hexyl, 100 x 2.1 mm, 2.7 μm |
Mobile Phase A |
5 mM ammonium formate in water |
Mobile Phase B |
5 mM ammonium formate in methanol |
Mobile Phase
Gradient |
Start at 10% mobile phase B and hold it for 1 min.,
then increase B to 95% in 15 min. and keep at 95% B for 2 min.
Finally equilibrate the column at initial condition for 3 min. |
Column Oven
Temperature |
40 °C |
Auto Sampler
Temperature |
15 °C |
Injection Volume |
1.0 μL |
MS Source Conditions |
ESI Voltage (Positive) |
5000 V |
ESI Voltage (Negative) |
-4000V |
Drying Gas |
140 |
Nebulizer Gas |
350 |
Source Temperature |
325 °C |
HSID Temperature |
200 °C |
Detection mode |
Time-managed MRM™ |
Table 2. MRM Transitions (partial list of the 213 pesticides studied). Source: PerkinElmer Food Safety and Quality
Compound
Name |
Polarity |
Q1
Mass |
Q2
Mass |
CE |
EV |
CCL2 |
Acephate |
Positive |
184.1 |
143.1 |
-12 |
25 |
-29 |
Acephate-2 |
Positive |
184.1 |
125.1 |
-25 |
25 |
-41 |
Acetamiprid |
Positive |
223.2 |
126.1 |
-30 |
25 |
-49 |
Acetamiprid-2 |
Positive |
223.2 |
99.1 |
-56 |
25 |
-73 |
Azoxystrobin |
Positive |
404.1 |
372.1 |
-18 |
25 |
-57 |
Azoxystrobin-2 |
Positive |
404.1 |
344.1 |
-34 |
25 |
-71 |
Buprofezin |
Positive |
306.2 |
201.1 |
-18 |
25 |
-47 |
Buprofezin-2 |
Positive |
306.2 |
116.2 |
-24 |
25 |
-52 |
Chlorantranilprole |
Positive |
484 |
452.8 |
-20 |
25 |
-66 |
Chlorantranilprole-2 |
Positive |
484 |
285.8 |
-18 |
25 |
-65 |
Chlorpyriphos |
Positive |
350 |
198 |
-20 |
25 |
-53 |
Chlorpyriphos-2 |
Positive |
350 |
97 |
-32 |
25 |
-64 |
Clothianidin |
Positive |
250.1 |
169.1 |
-16 |
25 |
-39 |
Clothianidin -2 |
Positive |
250.1 |
132.2 |
-26 |
25 |
-48 |
Cumyluron |
Positive |
303.1 |
185 |
-20 |
25 |
-48 |
Cumyluron-2 |
Positive |
303.1 |
125 |
-43 |
25 |
-69 |
Fenbutatin-
oxide |
Positive |
519.3 |
197 |
-67 |
25 |
-112 |
Fenbutatin-oxide-2 |
Positive |
519.3 |
350.9 |
-50 |
25 |
-97 |
Fenobucarb |
Positive |
208 |
152 |
-12 |
25 |
-32 |
Fenobucarb-2 |
Positive |
208 |
95 |
-19 |
25 |
-38 |
Fluopyram |
Positive |
397 |
173 |
-35 |
25 |
-71 |
Fluopyram-2 |
Positive |
397 |
145 |
-70 |
25 |
-103 |
Halofenozide |
Positive |
331.1 |
275 |
-18 |
25 |
-49 |
Halofenozide-2 |
Positive |
331.1 |
104.9 |
-25 |
25 |
-56 |
Imazalil |
Positive |
297.1 |
201 |
-25 |
25 |
-52 |
Imazalil-2 |
Positive |
297.1 |
159.2 |
-31 |
25 |
-58 |
Imidachloprid |
Positive |
256.2 |
175.2 |
-26 |
25 |
-49 |
Imidachloprid-2 |
Positive |
256.2 |
209 |
-18 |
25 |
-42 |
Isoprothiolane |
Positive |
291.1 |
231 |
-16 |
25 |
-44 |
Isoprothiolane-2 |
Positive |
291.1 |
189 |
-28 |
25 |
-54 |
Malathion |
Positive |
331.1 |
127.1 |
-22 |
25 |
-53 |
Malathion-2 |
Positive |
331.1 |
99.1 |
-24 |
25 |
-55 |
Methamidophos |
Positive |
142 |
124.9 |
-20 |
25 |
-32 |
Methamidophos-2 |
Positive |
142 |
94.1 |
-20 |
25 |
-32 |
Piperonyl butoxide |
Positive |
356.2 |
177 |
-13 |
25 |
-47 |
Piperonyl butoxide-2 |
Positive |
356.2 |
119 |
-37 |
25 |
-69 |
Pirimiphos-methyl |
Positive |
306.1 |
164.1 |
-28 |
25 |
-56 |
Pirimiphos-methyl-2 |
Positive |
306.1 |
108.1 |
-40 |
25 |
-67 |
Profenophos |
Positive |
375 |
304.8 |
-50 |
25 |
-75 |
Profenophos-2 |
Positive |
375 |
346.8 |
-42 |
25 |
-113 |
Propiconazole |
Positive |
342.1 |
159.1 |
-42 |
25 |
-72 |
Propiconazole-2 |
Positive |
342.1 |
69.1 |
-26 |
25 |
-58 |
Tebuconazole |
Positive |
308 |
70 |
-30 |
25 |
-58 |
Tebuconazole-2 |
Positive |
308 |
125 |
-50 |
25 |
-76 |
Thiamethoxam |
Positive |
292 |
181 |
-28 |
25 |
-54 |
Thiamethoxam-2 |
Positive |
292 |
211 |
-18 |
25 |
-45 |
Triazophos |
Positive |
314.1 |
161.9 |
-22 |
25 |
-51 |
Triazophos-2 |
Positive |
314.1 |
118.9 |
-50 |
25 |
-76 |
Tricyclazole |
Positive |
190 |
163 |
-28 |
25 |
-44 |
Tricyclazole-2 |
Positive |
190 |
136 |
-36 |
25 |
-51 |
Trifloxystrobin |
Positive |
409 |
186 |
-26 |
25 |
-64 |
Trifloxystrobin-2 |
Positive |
409 |
206 |
-20 |
25 |
-59 |
Fludioxonil |
Negative |
246.6 |
125.9 |
40 |
-25 |
60 |
Fludioxonil-2 |
Negative |
246.6 |
179.9 |
39 |
-25 |
60 |
Results and Discussion
Analytical Challenges for Multi-Residue Pesticides Analysis in Food Samples
Since the pesticides investigated in this study contain both polar and non-polar compounds, the organic solvent acetonitrile was used to extract all analytes from the sample matrices. However, in the reverse phase LC method, an aqueous mobile phase was used at the start of the LC run to preserve the polar compounds on the column.
Injecting a greater volume of an organic solvent, such as an acetonitrile sample extract, onto the LC would result in diminished chromatographic peaks for early eluting polar compounds.
To tackle this problem, a small sample volume was injected in this study. Standard MRM method development is not appropriate for the analysis of a large number of analytes such as hundreds of pesticide residues in a single run. It is both time-consuming and labor-intensive to manually input all mass transitions into a method.
The dwell time for each transition cannot be easily optimized using conventional methods. Therefore, in this study, a time-controlled MRM was applied for method development to enhance efficiency, data quality and performance of the method.
The sample matrix effect is the primary concern when developing the LC/MS/MS method, particularly for food analysis due to the diversity and complexity of food sample matrices.
Various approaches have been used to overcome sample matrix effects, these include sample dilution, use of stable isotope internal standards, matrix-matched calibration, standard addition, sample clean-up, use of high-efficiency columns for better separation, and the use of alternative ionization sources.11
During this study, sample matrix effects were assessed by comparing the slopes (X) of calibration curves acquired from the standards prepared in solvent (neat solution) with slopes (Y) captured from standards prepared in the rice sample matrix. Sample matrix effect (%) can be quantified by the percentage difference between the slopes, i.e. (Y-X) × 100/X.
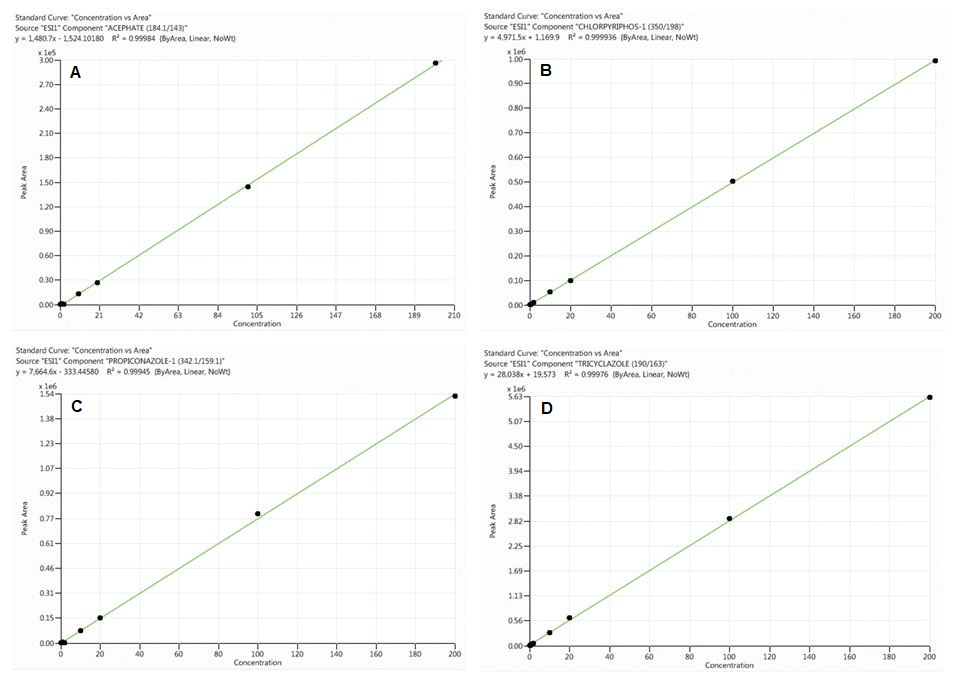
Figure 1. Calibration curves for acephate (A), chlorpyriphos (B), propiconazole (C) and tricyclazole (D) obtained from standards prepared in neat solutions
(analyte concentrations range from 0.1 to 200 ng/mL). Image Credit: PerkinElmer Food Safety and Quality
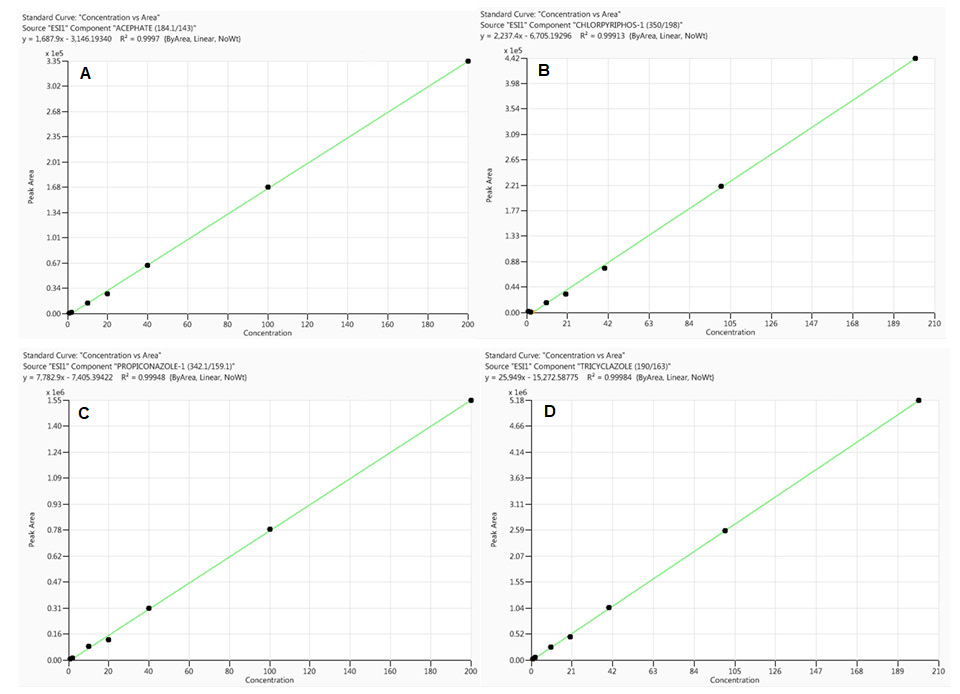
Figure 2. Calibration curves for acephate (A), chlorpyriphos (B), propiconazole (C) and tricyclazole (D) obtained from standards prepared in rice sample matrix (analyte concentrations range from 0.1 to 200 ng/mL). Image Credit: PerkinElmer Food Safety and Quality
When the percentage of the difference between the slopes of the two curves is positive, there is a signal enhancement effect, whereas a negative value indicates signal suppression effect. As shown in Table 3 and Figures 1 and 2, sample matrix effects are compound-dependent.
For example, some pesticides, such as acephate and propiconazole, demonstrated signal amplification (positive values), while others, such as chlorpyriphos and tricyclazole, demonstrated ion suppression (negative values).
As illustrated in Table 3, sample matrix effects for a majority of the pesticides under evaluation are less than 20% and thus, calibration curves made from neat solutions could be used for their quantification without any considerable error in compliance with EU regulation.12
However, serious ion suppression effects were witnessed for chlorpyriphos (-55%) and tebuconazole (-18%). Therefore, to bypass matrix effects and limit any variations in analytical results, matrix-matched calibrations were utilized throughout this study for the quantification of all analytes.
Table 3. Results of retention time, recovery, reproducibility (%RSD), matrix effect and linearity for the most commonly detected pesticides in rice samples. Source: PerkinElmer Food Safety and Quality
Pesticide |
Retention
Time (min) |
% Recovery (%RSD)
at 10 μg/kg |
% Recovery (%RSD)
at 100 μg/kg |
Matrix
Effect (%) |
Correlation
Coefficient (R2) |
Acephate |
1.88 |
101.1 (11.8) |
81.9 (4.3) |
14.0 |
0.9997 |
Acetamiprid |
8.15 |
106.5 (2.6) |
98.7 (2.3) |
2.7 |
0.9996 |
Buprofezin |
15.05 |
103.3 (2.9) |
98.8 (3.5) |
-3.1 |
0.9996 |
Chlorpyriphos |
15.54 |
109.6 (10.4) |
98.7 (5.0) |
-55.0 |
0.9991 |
Clothianidin |
6.70 |
105.7 (5.9) |
111.2 (8.6) |
17.0 |
0.9995 |
Cumyluron |
12.74 |
98.9 (7.2) |
96.1 (2.5) |
-2.6 |
0.9984 |
Fenbutatin-oxide |
16.90 |
69.5 (18.6) |
78.8 (12.7) |
13.1 |
0.9997 |
Fenobucarb |
11.20 |
101.6 (2.9) |
94.8 (1.9) |
2.6 |
0.9976 |
Fluopyram |
13.00 |
104.8 (3.6) |
101.1 (3.1) |
-2.7 |
0.9991 |
Halofenozide |
12.26 |
89.4 (15.2) |
88.3 (11.4) |
-4.4 |
0.9980 |
Imazalil |
14.33 |
89.6 (13.6) |
95.3 (4.1) |
-6.1 |
0.9996 |
Imidacloprid |
7.57 |
77.5 (10.8) |
112.2 (7.9) |
-5.7 |
0.9991 |
Isoprothiolane |
13.01 |
111.5 (2.7) |
101.1 (2.3) |
-0.4 |
0.9983 |
Malathion |
13.25 |
92.0 (12.0) |
86.0 (4.3) |
-9.9 |
0.9995 |
Methamidophos |
1.41 |
82.8 (10.1) |
76.4 (14.3) |
13.3 |
0.9978 |
Piperonyl Butoxide |
15.26 |
106.0 (5.0) |
105.2 (3.4) |
-6.3 |
0.9977 |
Pirimiphos-methyl |
14.71 |
107.5 (3.7) |
98.8 (5.3) |
-0.1 |
0.9997 |
Profenophos |
14.82 |
110.7 (6.9) |
103.0 (6.5) |
-2.5 |
0.9988 |
Propiconazole |
14.32 |
106.6 (7.1) |
98.3 (2.8) |
1.5 |
0.9994 |
Tebuconazole |
13.72 |
102.2 (6.9) |
104.2 (5.5) |
-18.9 |
0.9993 |
Thiamethoxam |
6.43 |
116.4 (10.0) |
114.0 (14.9) |
1.9 |
0.9991 |
Triazophos |
13.46 |
117.8 (5.7) |
99.5(3.0) |
2.7 |
0.9979 |
Tricyclazole |
9.27 |
84.2 (5.8) |
80.7 (7.8) |
-7.5 |
0.9998 |
Trifloxystrobin |
14.91 |
106.7 (2.4) |
106(4) |
-5.8 |
0.9991 |
Method Performance
All calibration curves made from both the neat solution and rice sample matrix (matrix-matched calibration) demonstrated excellent linearity (0.1 to 200 ng/mL) with correlation coefficient (R²) greater than 0.99 (see Figures 1 and 2 for basic examples of calibration curves).
The recoveries of pesticides were assessed by spiking the analytes to the samples at two concentration levels of 10 and 100 μg/kg, respectively.
As shown in Table 3, the recoveries of analytes ranged from 70% to 120% with RSD <20% for a majority of the pesticides studied. The limits of quantification (LOQs) were established by considering the signals of both quantifier and qualifier ions (S/N > 10 for both) and making sure that the product ion ratios were within 20% tolerance windows of the expected.12
A majority of the pesticides tested possess LOQs ranging from 0.5 to 20 µg/kg, which are well below the EU MRLs.
Sample Analysis
The method developed was used for the analysis of pesticide residues in various food samples, including 11 rice samples; one wheat sample and one veggie straw sample.
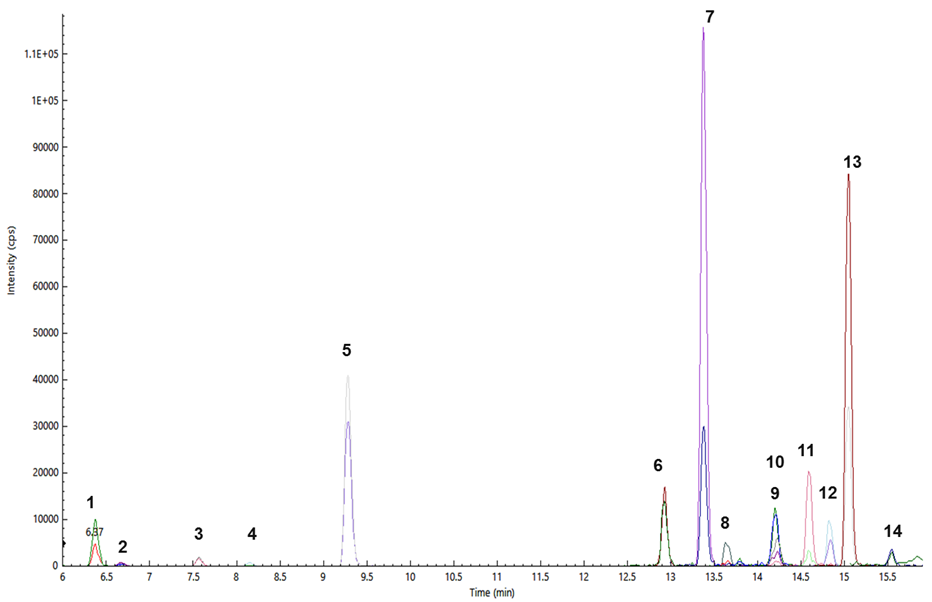
Figure 3. Pesticides determined from a brown rice sample (S10): thiamethoxam (1), clothianidin (2), imidacloprid (3), acetamiprid (4), tricyclazole (5), isoprothiolane (6), triazophos (7), tebuconazole (8), imazalil (9), propiconazole (10), profenophos (11), trifloxystrobin (12), buprofezin (13), and chlorpyriphos (14). Image Credit: PerkinElmer Food Safety and Quality
Figure 3 shows the overlapped MRM chromatograms of pesticides identified and quantified from a brown rice sample. Table 4 records the pesticide residues identified in the 11 rice samples and the EU MRLs in µg/kg (NA*; some pesticides that are not covered by the EU MRLs list were also found using this method).
As illustrated in Table 4, several of the pesticides determined from sample 4 (S4) and sample 10 (S10) are rather similar due to the fact that these two rice samples were grown in the same region, which implies that pesticides applied to rice crops throughout production are region- or country-specific due to the regulation and weather conditions in that area.
Table 4. Pesticide residues determined from eleven rice samples (S1 to S11), in μg/kg. Source: PerkinElmer Food Safety and Quality
Pesticide |
S1 |
S2 |
S3 |
S4 |
S5 |
S6 |
S7 |
S8 |
S9 |
S10 |
S11 |
MRL |
Acephate |
|
|
|
2.0 |
|
|
|
|
|
|
|
10 |
Acetamiprid |
|
|
|
|
|
|
0.3 |
|
|
0.8 |
|
10 |
Buprofezin |
|
|
|
9.1 |
|
|
|
|
|
46.5 |
|
500 |
Chlorpyriphos |
|
|
|
0.5 |
0.3 |
|
1.4 |
|
|
8.7 |
|
50 |
Clothianidin |
|
|
|
7.0 |
|
|
|
|
|
3.0 |
|
500 |
Fenobucarb |
|
|
|
4.1 |
|
|
|
|
|
|
|
NA* |
Fluopyram |
|
|
|
|
0.5 |
|
|
|
|
|
|
10 |
Halofenozide |
|
|
|
|
|
5.0 |
|
|
|
|
|
NA* |
Imazalil |
1.4 |
|
|
2.5 |
|
4.6 |
|
|
|
2.6 |
1.6 |
50 |
Imidacloprid |
|
|
|
2.8 |
|
|
1.1 |
|
|
9.2 |
|
1500 |
Isoprothiolane |
|
|
|
4.4 |
9.3 |
|
2.9 |
|
|
14.7 |
|
5000 |
Malathion |
|
|
|
|
|
|
1.8 |
|
2.2 |
|
|
8000 |
Methamidophos |
|
|
|
0.5 |
|
|
|
|
|
|
|
10 |
Piperonyl Butoxide |
|
0.6 |
1.3 |
|
|
|
|
0.8 |
|
|
|
NA* |
Pirimiphos-methyl |
|
|
1.4 |
|
|
|
|
|
|
|
|
500 |
Profenophos |
|
|
|
|
|
|
|
|
|
5.2 |
|
10 |
Propiconazole |
|
|
|
8.3 |
8.4 |
6.7 |
4.1 |
|
|
18.1 |
|
1500 |
Tebuconazole |
|
|
|
5.9 |
5.2 |
|
0.9 |
|
|
12.0 |
|
1000 |
Thiamethoxam |
|
|
|
10.6 |
|
|
|
|
|
11.0 |
|
10 |
Triazophos |
|
|
|
0.6 |
|
|
0.5 |
|
|
17.6 |
|
20 |
Tricyclazole |
|
|
16.4 |
5.8 |
7.6 |
20.6 |
0.6 |
|
|
40.2 |
|
1000 |
Trifloxystrobin |
|
|
|
|
|
|
|
|
|
1.6 |
|
5000 |
NA*: pesticides not listed in the EU MRLs database, but can be determined by this method.
Conclusion
By coupling a UHPLC system to a QSight 220 triple-quad mass spectrometer, a LC/MS/MS method was developed for multi-residue pesticides analysis in rice.
The method can be used for the analysis of more than 200 pesticides in rice with LOQs well below the regulatory limits.
The time-managed-MRM module has streamlined the production of MS method with the ideal dwell time for evaluating many analytes in food samples.
The QuEChERS sample extraction used for this study showed good recovery (70-120%) and reproducibility (RSD 0.99) for all the pesticides studied in the rice matrix.
Various pesticide residues were established and quantified from eleven rice samples with concentrations at or below the EU MRLs.
This LC/MS/MS method has also been utilized for other food analyses such as wheat and veggie strews samples with excellent performance. The method shown here can be adapted easily for multianalyte screening and quantification, offering a single method for cost-effective analysis of pesticides in rice and other food samples.
References
- Commission Regulation (EC) 396/2005 on maximum residue levels of pesticides in or on food and feed of plant and animal origin, J. Eur. Union. L70/1 (2005).
- U.S. Environmental Protection Agency, Electronic code of federal regulation: Title 40: part 180-tolerance and exemptions for pesticide chemical residues in Food. https://www.ecfr.gov/
- China National Standard GB 28260-2011. 2011. Maximum residue limits for 85 pesticides in food, Ministry of Health of the People’s Republic of China.
- X. Hou, M. Han, X. Dai, X-F. Yang and S. Yi, A multi- residue method for the determination of 124 pesticides in rice by modified QuEChERS extraction and GC-MS/MS. Food Chemistry, 2013, 138, 1198-1205.
- M. Kirchner, E. Matisova, S. Hrouzkova, and J. D. Zeeuw, Possibilities and limitations of quadrupole mass spectrometric detector in fast gas chromatography. J. Chromatogr. A, 2005, 1090 (1-2), 126–132.
- J. Wu, Quantitative Method for the Analysis of Tobacco-Specific Nitrosamines in Cigarette Tobacco and Mainstream Cigarette Smoke by Use of Isotope Dilution Liquid Chromatography Tandem Mass Spectrometry, Anal. Chem., 2008, 80 (4), 1341–1345.
- K. Zhang, M.R. Schaab, G. Southwood, E.R. Tor, L.S. Aston, W. Song, B. Eitzer, S. Majumdar, T. Lapainus, H. Mai, K. Tran, A. El-Demerdash, V. Vega, Yanxuan Cai, J.W. Wong, A.J. Krynitsky, and T.H. Begley, A Collaborative Study: Determination of Mycotoxins in Corn, Peanut Butter, and Wheat Flour Using Stable Isotope Dilution Assay (SIDA) and Liquid Chromatography–Tandem Mass Spectrometry (LC-MS/MS), J. Agric. Food Chem. 2017, 65 (33), 7138-7152.
- A. Wilkowska and M. Biziuk, Determination of pesticide residues in food matrices using the QuEChERS methodology, Food Chemistry, 2011, 125, 803-812.
- L. Pareja, A.R. Fernandez-Alba, V. Cesio and H. Heinzen, Analytical methods for pesticide residues in rice, Trends in Anal. Chem. 2011, 30 (2), 270-291.
- L. Pareja, V. Cesio, H. Heinzen and A.R. Fernandez-Alba, Evaluation of various QuEChERS based methods for the analysis of herbicides and other commonly used pesticides in polished rice by LC-MS/MS, Talanta, 2011, 83, 1613-1622.
- A. J. Krynitsky, J. W. Wong, K. Zhang and H. Safarpour, Focus on Food Analysis: Important considerations regarding matrix effects when developing reliable analytical residue methods using mass spectrometry, LCGC North America, 2017,Vol. 35, No. 7, 444-451.
- European Commission, SANCO. 2015. Guidance document on analytical quality control and method validation procedures for pesticides residues analysis in food and feed, SANTE/11945/2015 https://www.eurl-pesticides.eu/docs/public/tmplt_article.asp?CntID=727.
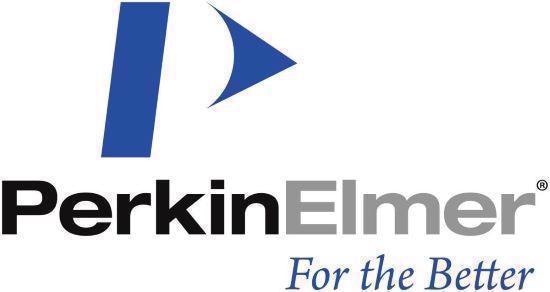
This information has been sourced, reviewed and adapted from materials provided by PerkinElmer Food Safety and Quality.
For more information on this source, please visit PerkinElmer Food Safety and Quality.