Oct 25 2021
Researchers are investigating how to substitute the PZT materials to protect the environment. Continuous research is going on to build up different lead-free materials, for eco-friendly applications. This article considers research conducted by Kuanar, B et al.
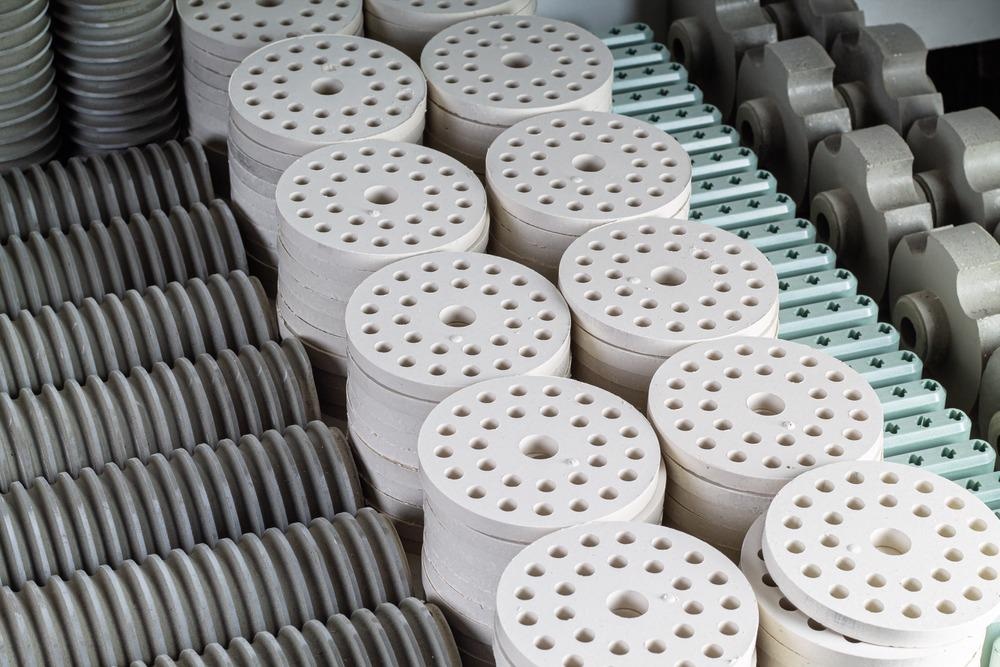
Image Credit: BossCo77/Shutterstock.com
Scientists have been focusing on the application of high dielectric constant capacitors, buzzers, sensors, ultrasonic motors, actuators, piezoelectric sonar, ultrasonic transducers, medical diagnostic transducers, ferroelectric thin films memories, multilayer capacitors, ferroelectric random-access memory (FERAM), and so on. Piezoceramic PZT used in functional devices is toxic due to the presence of lead.
At present researchers are diverted towards lead-free piezoelectric ceramics, namely titanate-based perovskite material (BaTiO3, Na0.5Bi0.5TiO3, K0.5Bi0.5TiO3, and BaZr0.08Ti0.92O3), and its solid solutions.
The main drawback of natural piezoelectric materials is truncated piezoelectricity. Lead oxide is a PZT component and is highly toxic in behavior. The toxicity can be enhanced because of its volatility at a high temperature.
Between the two different lead-free systems: (i) Perovskite materials are Bismuth Sodium Titanate (BNT), Barium Titanate Oxide (BaTiO3), Potassium Niobate (KNbO3), Sodium Titanate Oxide (NaTiO3), etc. (ii) Non-Perovskite materials such as Bismuth layer structure and Tungsten-Bronze have appeared as a suitable material for the substitution of the lead-based ceramics.
However, the main bottleneck includes low Curie temperature (TC) and high coercive field that make the ceramic strenuous for polarization state transition with remarkable conductivity and dielectric loss.
Due to the large remnant polarization (Pr∼38 μC/cm2), Bismuth sodium titanate (Ba0.5Na0.5TiO3; BNT) is assumed as a righteous candidate of lead-free piezoelectric ceramics at room temperature. BNT holds a complex perovskite structure and rhombohedral phase. If the transition temperature is 200 °C, it changes to the anti-ferroelectric phase. The Curie temperature (Tc) of such material is about 320 °C.
Because of the above advantages of BNT, the authors mainly focused on the changes in the transition of phases, electrical and dielectric behaviors of BNT, its solid solutions with other perovskite structures, and doping of di, tri, and tetravalent ions at A and/or B-site.
Methodology
The sample stoichiometric followed by various research groups is displayed in Figure 1. Out of various powder preparation methods available, most commonly the mixed oxide route is followed where appropriate stoichiometric proportions of desired oxides are used. To achieve the required chemical and optical homogeneity, ball milling is crucial. Milling time is based on powder characteristics and varies from 2 to 16 hours.
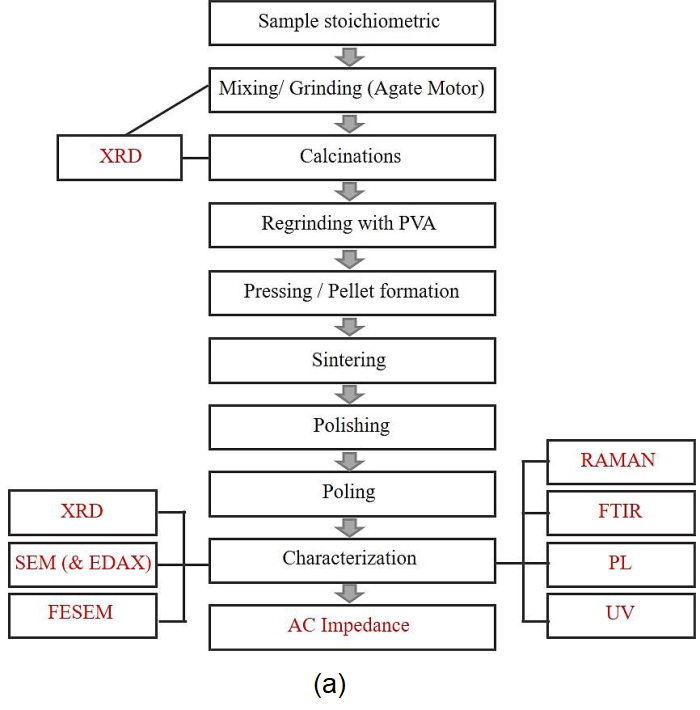
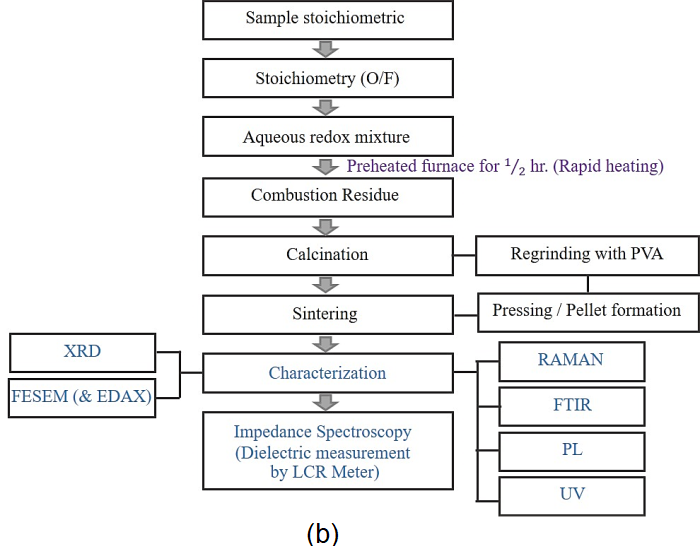
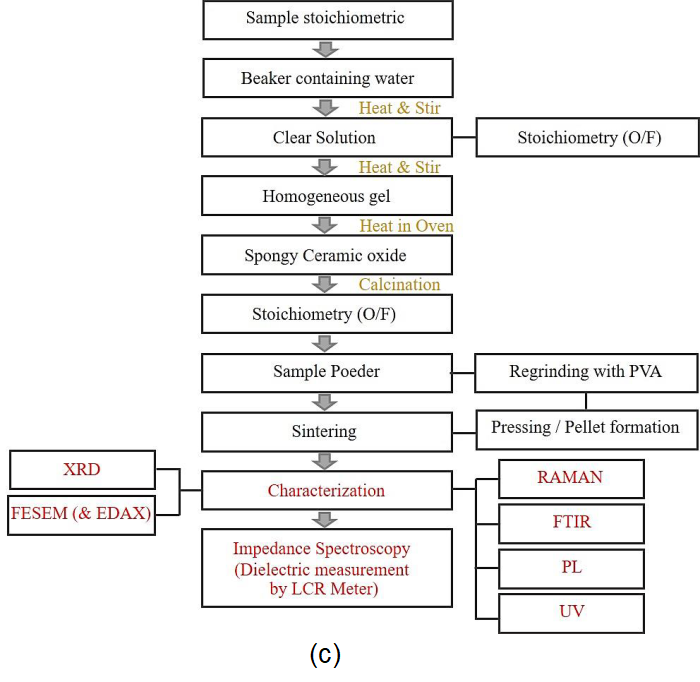
Figure 1. Steps for sample preparation and characterization: (a) Solid-state route, (b) Auto-combustion route, and (c) Sol-gel route. Image Credit: Kuanar, et al., 2021
Generally, the mixtures are milled with either zirconia or alumina balls with non-flammable liquid-like trichloroethylene or distilled water. The milled powders are then dried and calcined (800 °C–900 °C) for 2 to 3 hours.
During calcination, volatile components like moisture are separated and then the required phase formation occurs. The organic vehicle generally includes a binder (ethylcellulose), a plasticizer (polyethylene glycol), a dispersing agent (butoxy ethoxy ethyl acetate), and a solvent (α-terpineol).
After the calcination process, polyvinyl alcohol (PVA) is used as a binder to press the granulated powder pellets. The pellets are then sintered at a temperature varying between 1050 °C-1200 °C. For the electrode, the sintered pellets are coated with silver paste, and for orientation, the ferroelectric domains in ceramic and electrical properties are measured by applying a strong DC field.
Dielectric measurement (dielectric loss and dielectric constant) was performed with the use of an automatic LCR Meter Bridge. A Sawyer-Tower circuit is used to perform ferroelectric hysteresis measurement. A constant tester of the piezoelectric is used to measure the piezoelectric coefficient d33. The other properties of materials are studied by using Raman, FTIR, PL, and UV by the researchers for various industrial applications.
Results and Discussion
The ferroelectric oxides with general formula ABO3, considered as a perovskite structure, such as Bi0.5Na0.5TiO3(BNT), BaTiO3(BT), KNbO3, NaTiO3, NaTaO3, etc. are the renowned lead-free piezoelectric materials. The physical properties of such structure are tuned easily with the use of several iso- and aliovalent substitutions either at A site or B site of ABO3 structure as per the requirement of its simple structure.
It has been observed that BNT-based compositions modified with BaTiO3, BiKTiO3, NaNbO3, BiFeO3, La2O3, Sc2O3, BaCO3, etc. showed improved properties and are easy to be poled.
The substitution of Zr in the B-site enhances the relative ionic displacement and electrical property by the expansion of the perovskite lattice. It can also be seen that the substitution of Zr suppresses the conduction in electronic hoping in an oxidation state of Ti4+ and Ti3+.
The addition of Gd at the Bi site shows distortion, and it is an amphoteric dopant. Therefore, there is a possibility of using this material as a photovoltaic cell.
Bismuth magnesium titanate (Bi (Mg0.5Ti0.5) O3) [BMT] is a rhombohedral ferroelectric perovskite similar to sodium bismuth titanate (Bi0.5Na0.5) TiO3 [BNT] ceramics. For improving the ferroelectric and piezoelectric properties, researchers have studied the Mg dopant’s effects as an additive. It has been concluded that the Rhombohedral structure is maintained till x = 0.04-mole fraction, and it changed to a cubic phase in higher mole fraction.
Piezoelectric and thermal depoling properties of Bismuth Sodium Titanate ceramic were investigated with a new group of compounds (Bi0.5Na0.5) TiO3-(Bi0.5(1+x) Na0.5) TiO3-0.75x. The ratios of assumed and measured densities were more than 97%. With the increase in Bi or decrease in Na in BNT, the resistivity increases.
Another interesting BNT based ceramic (1-x) NBT-xBT was studied by researchers. The transition of structural phase using XRD and Raman spectra with intermediate coexistence of the morphotropic phase boundary (MPB) has been reported. All the ferroelectric and dielectric properties are enlisted in Table 1.
Table 1. Properties of NBT-BT system. Source: Kuanar, et al., 2021
Compositions
(1-x) NBT-xBT |
Tm (°C) |
TFR (°C) |
tanδ |
εr |
Pr (μC/cm2) |
Ec (kV/cm) |
x = 0.00 |
335 |
170 |
0.01389 |
2100 |
71.8 |
58.6 |
x = 0.02 |
315 |
160 |
0.00571 |
2500 |
46.7 |
48.2 |
x = 0.05 |
305 |
137 |
0.01229 |
3800 |
15.4 |
34.7 |
x = 0.06 |
290 |
105 |
0.0355 |
4800 |
57.7 |
28.8 |
x = 0.07 |
270 |
109 |
0.04749 |
4100 |
37.1 |
22.1 |
x = 0.08 |
260 |
120 |
0.06313 |
2900 |
17.1 |
22.4 |
x = 0.10 |
255 |
133 |
0.0193 |
2400 |
13.94 |
22.6 |
The influence of a small amount of (2.0 wt.%) of Ba2+ ions on A- and B-site substitution in BNT was studied. With the increase in frequency, there was a rapid decrease in the dielectric constant. The dielectric loss reduces with the increasing frequency.
The highest piezoelectric coefficient (d33) of 1658 pC/N and large electrostrictive coefficient Q = 0.287 m4C-2 were obtained in the ceramics of (1-x) Bi0.5Na0.5TiO3-xSr0.85Bi0.1TiO3 sintered at 1260 °C and most importantly the P-E loops were hysteresis-free, indicating a suitable material for high-precision actuator application.
When x is closed to 0.06-0.08, the presence of MPB is revealed in Figure 2 reflecting binary system phase (1-x) Bi1/2Na1/2TiO3–x BaTiO3 (BNT-BT). Based on the enhanced property of piezoelectric, MPB existence becomes evident at this composition.
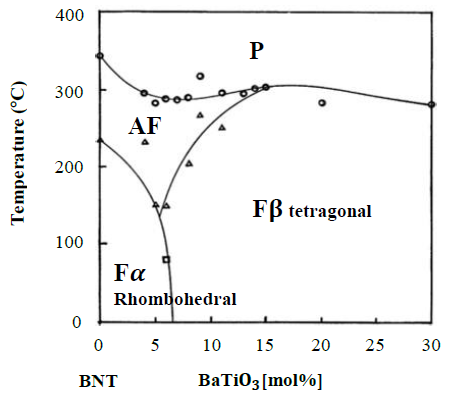
Figure 2. Morphotropic Phase boundaries in BNT-BT. Image Credit: Kuanar, et al., 2021
Researchers did a further investigation on the dielectric and ferroelectric properties of BNT-BT-xBZT (with 0 ≤ x (BZT) ≤ 0.10). They synthesized the ceramic by the traditional solid-state reaction method.
They also confirmed the co-existence of rhombohedral and tetragonal phases for all samples, and that the tetragonal phase increased with an increasing amount of BZT. In Table 2, the values of dielectric constant (εr), dielectric loss (tanδ), phase transition temperature (TFA), remnant polarization (ps), and coercive field (Ec) are presented.
Table 2. Properties of BNT-BT-xBZT system. Source: Kuanar, et al., 2021
Compositions (x)
[(0.935-x) BNT-0.065BT-xBZT] |
εr
at TFA |
tanδ
at TFA |
TFA |
TSA |
Pr
(μC/cm2) |
Ec
(kV/cm) |
x = 0 |
6820 |
0.015 |
148 |
265 |
32 |
30 |
x = 0.02 |
6956 |
0.015 |
154 |
268 |
29 |
23 |
x = 0.04 |
7058 |
0.011 |
143 |
280 |
24 |
20 |
x = 0.06 |
6913 |
0.011 |
181 |
263 |
24 |
26 |
x = 0.08 |
7234 |
0.014 |
197 |
259 |
20 |
29 |
x = 0.10 |
8224 |
0.014 |
210 |
249 |
21 |
31 |
The role of BiFeO3 on the electrical and electrochemical properties as well as structure (microstructure) of the BNT-BKTx-BFy ternary system is presented in Table 3. The incorporated BF disseminates into the BNT-BKT lattice to come out a solid solution but decreases the tetragonal and rhombohedral distortions with a strong influence on properties concluding as a good piezoelectric material.
Table 3. Properties of BNT-BKTx-BFy system. Source: Kuanar, et al., 2021
Compositions (x,y) |
εr |
tan δ |
Pr (μC/cm2) |
Ec (kV/mm) |
d33 (pC/N) |
(0.18, 0) |
1344 |
0.047 |
33.5 |
3.20 |
150 |
(0.18, 0.03) |
1233 |
0.048 |
28.9 |
3.16 |
125 |
(0.18, 0.07) |
1187 |
0.052 |
27.5 |
2.73 |
70 |
(0.20, 0) |
1743 |
0.053 |
37.5 |
2.90 |
195 |
(0.20, 0.03) |
1411 |
0.053 |
33 |
2.36 |
155 |
(0.20, 0.07) |
1235 |
0.055 |
28.9 |
2.00 |
120 |
(0.22, 0) |
1518 |
0.051 |
34 |
1.64 |
160 |
(0.22, 0.03) |
1375 |
0.054 |
7.6 |
0.83 |
20 |
(0.22, 0.07) |
1274 |
0.056 |
5.4 |
0.78 |
8 |
Researchers also investigated morphotropic phase boundary in the BNT-BKT-BT system as shown in Figure 3. With the increase in BKT and BT amount, the value of the dielectric constant increases for all compositions. The ternary mixture, i.e., 0.865 BNT–0.035 BT–0.100 BKT morphotropic composition shows high electromechanical coupling factors (kp = 0.26 and kt = 0.57) piezoelectric constant (d33 = 133 pC/N) which confirm good piezoelectric property and is presented in Table 4.
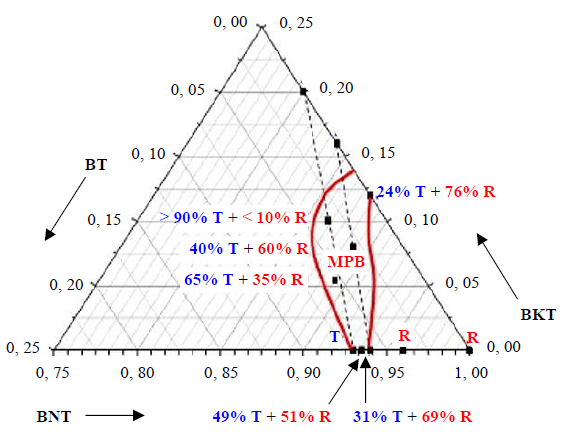
Figure 3. Morphotropic phase boundary of BNT-BKT-BT System. Image Credit: Kuanar, et al., 2021
Table 4. Properties of BNT-BKT-BT content. Source: Kuanar, et al., 2021
Compounds |
εr |
tanδ |
kp |
kt |
d33 (pC/N) |
BNT |
423 |
5.0 |
0.11 ± 0.01 |
0.49 ± 0.02 |
67 ± 10 |
0.96 BNT-0.04 BT |
532 |
1.7 |
0.13 ± 0.01 |
0.43 _± 0.02 |
123 ± 10 |
0.94 BNT-0.06 BT |
782 |
3.1 |
0.35 ± 0.02 |
0.53 ± 0.05 |
170 ± 4 |
0.935 BNT-0.065 BT |
873 |
3.4 |
0.26 ± 0.01 |
0.49 ± 0.05 |
147 ± 9 |
0.93 BNT-0.07 BT |
626 |
2.1 |
0.18 ± 0.02 |
0.45 ± 0.05 |
123 ± 3 |
0.88 BNT-0.12 BKT |
541 |
4.1 |
0.22 ±0.01 |
0.43 ± 0.03 |
78 ± 9 |
0.84 BNT-0.16 BKT |
710 |
3.6 |
0.29 ± 0.01 |
0.44 ± 0.02 |
130 ± 7 |
0.80 BNT-0.20 BKT |
936 |
3.9 |
0.39 ± 0.01 |
0.54 ± 0.05 |
137 ± 7 |
0.892 BNT-0.054 BT-0.054 BKT |
904 |
3.6 |
0.20 ± 0.02 |
0.43 ± 0.01 |
130 ± 10 |
0.89 BNT-0.03 BT-0.08 BKT |
665 |
3.8 |
0.26 ±0.01 |
0.44 ± 0.02 |
112 ± 4 |
0.865 BNT-0.035 BT-0.100 BKT |
891 |
4.2 |
0.26 ± 0.02 |
0.57 ± 0.05 |
133 ± 6 |
The result of NiNb2O6 content on the dielectric properties and structure of the BT-BNT system was observed. The result shows that doping of NiNb2O6 can suppress grain growth. Initially, the dielectric constant increased at a low temperature then decreased. Curie temperature has been increased slightly with doping concentration.
For 1.5 mol.% NiNb2O6 doped specimen, exceptional dielectric properties have been revealed at room temperature with a dielectric constant value 1652 and a dielectric loss value found in different pieces of literature, as mentioned in Table 5.
Table 5. Properties of some BNT-based solid solution systems. Source: Kuanar, et al., 2021
Compositions |
εr |
tanδ
(%) |
d33
(pC/N) |
Kp
(%) |
Tc
(℃) |
Td
(℃) |
Pr
μC/ cm2 |
Ref. |
(1-x) Na0.5Bi0.5TiO3-xBi (Mg0.5Ti0.5)O3 |
|
|
108 |
|
|
|
|
[52] |
(Bi0.5Na0.5) TiO3-(Bi0.5(1+x) Na0.5)TiO3-0.75x |
583 |
|
72.9, 81.3
(highest) |
|
|
187
(highest) |
|
[53] |
(1-x) Bi0.5Na0.5TiO3-xSr0.85Bi0.1TiO3 |
|
|
1658 |
|
|
|
|
[55] |
(K0.5Na0.5)1-3x BiNbO3 |
750 |
0.03 |
164 |
0.47 |
403 |
|
30.1 |
[56] |
0.8(Na0.5Bi0.5) TiO3-0.2(K0.5Bi0.5) TiO3 |
|
|
215 |
|
|
|
56.7 |
[57] |
NaBiTiZrO3(5%Zr) |
1618 |
|
|
|
313 |
|
|
[50] |
(Na0.5Bi0.5)1-x Bax TiO3 (6 mol%) |
787 |
0.0709 |
120 |
|
|
|
|
[59] |
0.92(Bi0.5Na0.5TiO3) +0.08 (BaTiO3) |
2573 |
<0.1 |
|
|
301 |
|
|
[60] |
0.912Ba0.97TiO3-0.088 (Bi0.5Na0.5) TiO3-xTa2O5 |
|
|
|
|
170 |
|
|
[61] |
0.93(Bi0.5Na0.5) TiO3-0.07 BaTiO3 |
|
|
151 |
0.278 |
|
|
|
[62] |
[(Bi0.5Na0.5)-TiO3]0.92-[BaTiO3]0.08 (BNT-BT0.08) |
243 |
|
|
|
|
|
0.87 |
[63] |
Bi0.465-xLax Na0.465 Ba0.07 TiO3 |
|
|
152 |
|
|
|
|
[65] |
(Bi1-xLax)0.5 Na0.5 TiO3 (3%La doped) |
|
|
209 |
|
365 |
|
27 |
[40] |
Bi0.5Na0.5(Ti1-xScx) O3 (5%Sc doped) |
|
|
155 |
|
370 |
|
20 |
[40] |
0.77Bi0.5Na0.5TiO3-0.20 Bi0.5K0.5TiO3-0.03 K0.5 Na0.5 NbO3 |
1460 |
|
164 |
|
|
|
125 |
[66] |
0.85Ba0.5Na0.5TiO3-0.11 Bi0.5K0.5-xRbxTiO3-0.04 BaTiO3 |
|
|
203 |
|
|
|
|
[70] |
0.9BaTiO3-0.1 (Bi0.5 Na0.5) TiO3+NiNb2O6 |
1652 |
1.8 |
|
|
144.9 |
|
|
[72] |
Conclusion
Lead-free ferroelectric ceramic oxides are highly used in modern technology for their high dielectric constant, piezoelectric coefficient, large remnant polarization, low coercive field, and high Curie temperature to substitute the extensively used lead-based ceramics, due to their pollution-free eco-friendly nature. However, these ferroelectric oxides suffer serious polarization problems.
Due to the large coercive field and high conductivity, PZT is ahead of BNT in industrial applications. For this reason, researchers intend to develop new lead-free ferroelectric oxides having suitable properties for multi-functional applications in a wide temperature and frequency range.
A novel type of application of the materials is the photostrictive actuator, which is the combined result of the photovoltaic effect and piezoelectric effect. One of the applications of piezoelectric composites is piezoelectric transducers. These ceramics-polymer composites have a broad range of applications in hydrophones, sensors and, medical ultrasonic.
Researchers have been investigating many dopants into bismuth sodium titanate ceramics to resolve industrial needs, and in the future it is expected that smart and very smart materials will be developed for a wide range of applications.
Journal Reference:
Kuanar, B., Mohanty, H. S., Behera, D., Nayak, P., Dalai, B. (2021) An elementary survey on structural, electrical, and optical properties of perovskite materials. Engineering and Applied Science Research. Available at: https://doi.org/10.14456/easr.2022.30.
References and Further Reading
Awan, M. Q., et al. (2018) Structure, dielectric and ferroelectric properties of lead free (K, Na) (Nb)O3-xBiErO3 piezoelectric ceramics. Journal of Materials Science: Materials in Electronics, 29(9), pp. 7142-7151. doi.org/10.1007/s10854-018-8702-3.
Hussain, A., et al. (2018) Giant piezoelectric behavior in relaxor ferroelectric environment friendly Na0.52K0.44Li0.04Nb0.84Ta0.10Sb0.06O3 ceramics for high temperature applications. Journal of Materials Science: Materials in Electronics, 29(8), pp. 6403-6411. doi.org/10.1007/s10854-018-8620-4.
Barick, B. K., et al. (2013) Dielectric and impedance spectroscopy of zirconium modified (Na0.5Bi0.5) TiO3 ceramics. Ceramics International, 39(5), pp. 5695-5704. doi.org/10.1016/j.ceramint.2012.12.087.
Reichmann, K., et al. (2015) Bismuth sodium titanate-based materials for piezoelectric actuators. Materials, 8(12), pp. 8467-8495. https://doi.org/10.3390/ma8125469.
Li, S., et al. (2020) Piezoelectricity and flexoelectricity of sodium bismuth titanate-based ceramics. Ceramics International, 46, pp. 2049-2054. https://doi.org/10.1016/j.ceramint.2019.09.184.
Pal, V., et al. (2013) Dielectric and ferroelectric properties of lead-free [1-{(Bi1-xLax)0.5(Na1-yLiy)0.5TiO3}-zBaTiO3] ceramic system. Advances in Materials Science and Engineering, 2013, p. 125634. https://doi.org/10.1155/2013/125634.
Anem, S., et al. (2016) Investigation of lanthanum substitution in lead-free BNBT ceramics for transducer applications. Ceramics International, 42(14), pp. 15319-15326. https://doi.org/10.1016/j.ceramint.2016.06.173.
Okayasu, M., et al. (2013) Material properties of bismuth layered ferroelectrics and lead zirconate titanate piezoelectric ceramics. Ceramics International, 39, pp. 3301-3306. https://doi.org/10.1016/j.ceramint.2012.10.018.
Perumal, R. N., et al. (2018) Influence of lead titanate additive on the structural and electrical properties of Na0.5Bi0.5TiO3-SrTiO3 piezoelectric ceramics. Ceramics International, 44(11), pp. 13259-13266. https://doi.org/10.1016/j.ceramint.2018.04.155.
Babu, M. V. G., et al. (2018) Coexistence of ferroelectric phases and electric field induced structural transformation in sodium potassium bismuth titanate ceramics. Journal of Applied Physics, 123(3), p. 234101. doi.org/10.1063/1.5021830.
Kornpom, C., et al. (2017) Enhancing piezoelectric of d33 coefficient of new (1-x) BNKLLT-xNKLNST lead-free ceramics synthesized by the solid state combustion technique. Integrated Ferroelectrics: An International Journal, 177(1), pp. 121-130. doi.org/10.1080/10584587.2017.1285663.
Barick, B. K., et al. (2011) Impedance and Raman spectroscopic studies of (Na0.5Bi0.5)TiO3. Journal of Physics D: Applied Physics, 44, p. 355402. https://doi.org/10.1088/0022-3727/44/35/355402.
Barick, B. K., et al. (2012) Phase transition and electrical properties of lanthanum-modified sodium bismuth titanate. Materials Chemistry and Physics, 132, p. 1007.
Mohanty, H. S., et al (2017) Studies of ferroelectric properties and leakage current behaviour of microwave sintered ferroelectric Na0.5Bi0.5TiO3 ceramic. Ferroelectrics, 517(1), pp. 25-33. doi.org/10.1080/00150193.2017.1369824.
Bajpai, K. K., et al. (2019) Cr-doped lead lanthanum zirconate titanate (PLZT) ceramics for pyroelectric and energy harvesting device applications. Ceramics International, 45(11), pp. 14111-14120. doi.org/10.1016/j.ceramint.2019.04.111.
Hiruma, Y., et al. (2008) Large electrostrain near the phase transition temperature of (Bi0.5Na0.5)TiO3-SrTiO3 ferroelectric ceramics. Applied Physics Letters, 92(26), p. 262904. doi.org/10.1063/1.2955533.
Zhu, Y., et al. (2019) Bipolar and unipolar fatigue property in Bi0.5Na0.5TiO3-Bi0.5K0.5TiO3-SrTiO3 lead-free piezoelectric ceramics. Physica B: Condensed Matter, 575, p. 411716. doi.org/10.1016/j.physb.2019.411716.
Lian, H. L., et al. (2018) Structure and electrical properties of Ca2+-doped (Na0.47Bi0.47Ba0.06)TiO3 lead-free piezoelectric ceramics. Ceramics International, 44(10), pp. 11320-11330. doi.org/10.1016/j.ceramint.2018.03.180.
Acosta, M., et al. (2015) Tailoring ergodicity through selective A-site doping in the Bi1/2Na1/2TiO3-Bi1/2K1/2TiO3 system. Journal of Applied Physics, 117(13), p. 134106. doi.org/10.1063/1.4916719.
Groh, C., et al. (2014) Frequency and temperature dependence of actuating performance of Bi1/2Na1/2TiO3-BaTiO3 based relaxor/ferroelectric composites. Journal of Applied Physics, 115(23), p. 234107. doi.org/10.1063/1.4876680.
Cho, J. H., et al. (2014) Phase transition and piezoelectric properties of lead-free (Bi1/2Na1/2)TiO3-BaTiO3 ceramics. Ceramics International, 40(6), pp. 8419-8425. https://doi.org/10.1016/j.ceramint.2014.01.051.
Dash, S. K., et al. (2014) Characterization and dielectric properties of barium zirconium titanate prepared by solid state reaction and high energy ball milling processes. Indian Journal of Physics, 88(2), pp. 129-135. doi.org/10.1007/s12648-013-0395-0.
Haertling, G H (1999) Ferroelectric ceramics: history and technology. Journal of the American Ceramic Society, 82(4), pp. 797-818. doi.org/10.1111/j.1151-2916.1999.tb01840.x.
Panda, P K (2009) Environmental friendly lead-free piezoelectric materials. Journal of Materials Science, 44, pp. 5049–5062. https://doi.org/10.1007/s10853-009-3643-0.
Lei, N., et al. (2011) Effect of lattice occupation behavior of Li+ cations on microstructure and electrical properties of (Bi1/2Na1/2)TiO3-based lead-free piezoceramics. Journal of Applied Physics, 109(5), p. 054102. doi.org/10.1063/1.3555598.
Xie, Z., et al. (1998) Microwave sintering of lead-based relaxor ferroelectric ceramics. Materials Letters, 36(1-4), pp. 191-194. doi.org/10.1016/S0167-577X(98)00024-X.
Badapanda, T., et al. (2013) Structure and dielectric properties of bismuth sodium titanate ceramic prepared by auto-combustion technique. Processing and Application of Ceramics, 7(3), pp. 135-141. doi.org/10.2298/PAC1303135B.
Szeremeta, A., et al. (2019) Improved piezoelectric properties of Pb(Zr0.70Ti0.30)O3 ceramics doped with non-polar bismuth manganite. Ceramics International, 45(15), pp. 18363-18370. doi.org/10.1016/j.ceramint.2019.06.051.
Mohanty, H. S., et al. (2018) Structural transformations and physical properties of (1-x) Na0.5Bi0.5TiO3-xBaTiO3 solid solutions near a morphotropic phase boundary. Journal of Physics Condensed Matter, 31(7), p. 075401. doi.org/10.1088/1361-648X/aaf405.
Du, H., et al. (2007) An approach to further improve piezoelectric properties of (K0.5Na0.5)NbO3-based lead-free ceramics. Applied Physics Letters, 91(20), p. 202907. doi.org/10.1063/1.2815750.
Mohanty, H. S., et al. (2018) Impedance spectroscopic study on microwave sintered (1-x) Na0.5Bi0.5TiO3-xBaTiO3 ceramics. Journal of Materials Science: Materials in Electronics, 29(8), pp. 6966-6977. doi.org/10.1007/s10854-018-8683-2.
Kornphom, C., et al. (2018) Enhanced dielectric and ferroelectric behavior in 0.94 BNT-0.06 BCTS lead free piezoelectric ceramics synthesized by the solid state combustion technique. Integrated Ferroelectrics: An International Journal, 187(1), pp. 20-32. doi.org/10.1080/10584587.2018.1445372.
Ullah, A., et al. (2020) Dielectric, ferroelectric, and strain properties of lead-free (1-y) BNT-y ST ceramics. Journal of Materials Science: Materials in Electronics, 31(6), pp. 5667-5673. doi.org/10.1007/s10854-020-03133-z.
Cui, W., et al. (2016) Large piezoelectric properties of (1-x) Na0.5Bi0.5TiO3-xBaTiO3 thin films prepared by sol-gel method. Journal of Materials Science: Materials in Electronics, 27(7), pp. 7287-7291. doi.org/10.1007/s10854-016-4696-x.
Razak, K. A., et al. (2016) Properties of Ce-doped Bi0.5Na0.5TiO3 synthesized using the soft combustion method. Procedia Chemistry, 19, pp. 816-821. doi.org/10.1016/j.proche.2016.03.107.
Zhao, Z. H., et al. (2019) Large electro-strain signal of the BNT-BT-KNN lead-free piezoelectric ceramics with CuO doping. Journal of Advanced Dielectrics, 9(3), p. 1950022. doi.org/10.1142/S2010135X1950022X.
Selvadurai, A. P., et al. (2015) Investigation of structural and optical spectroscopy of 5% Pr doped (Bi0.5Na0.5)TiO3 ferroelectric ceramics: site depended study. Journal of Materials Science: Materials in Electronics, 26, pp. 7655-7665.
Su Lee, D., et al. (2012) Electric field induced polarization and strain of Bi-based ceramic composites. Journal of Applied Physics, 112, p. 124109. doi.org/10.1063/1.4770372.
Huitema, L., et al. (2016) Microwave dielectric properties of BNT-BT0. 08 thin films prepared by sol-gel technique. Journal of Applied Physics, 119, p. 144103. doi.org/10.1063/1.4945822.
Verma, R & Rout, S K (2019) Frequency-dependent ferro-antiferro phase transition and internal bias field influenced piezoelectric response of donor and acceptor doped bismuth sodium titanate ceramics. Journal of Applied Physics, 126(9), p. 094103. doi.org/10.1063/1.5111505.
Suchanicz, J., et al. (2011) Raman and infrared spectroscopy of Na0.5Bi0.5TiO3-BaTiO3 ceramics. Journal of Electroceramics, 27(2), pp. 45-50. doi.org/10.1007/s10832-011-9648-5.
Hung, N. T., et al. (2018) Structural, optical, and magnetic properties of SrFeO3-d-modified Bi0.5Na0.5TiO3 materials. Physica B Condensed Matter, 531, pp. 75-78. https://doi.org/10.1016/j.physb.2017.12.021.
Cho, J. H., et al. (2014) Phase transition and piezoelectric properties of lead-free (Bi1/2Na1/2)TiO3-BaTiO3 ceramics. Ceramics International, 40(6), pp. 8419-8425. doi.org/10.1016/j.ceramint.2014.01.051.
Jan, S. U., et al. (2014) Electrical properties of Ca-modified Na0.5Bi0.5TiO3-BaTiO3 ceramics. Ceramics International, 40(10), pp. 15439-15445. https://doi.org/10.1016/j.ceramint.2014.06.107.
Parija, B., et al. (2013) Morphotropic phase boundary and electrical properties of 1-x [Bi0.5Na0.5]TiO3-xBa[Zr0.25Ti0.75]O3 lead-free piezoelectric ceramics. Ceramics International, 39(5), pp. 4877-4886. doi.org/10.1016/j.ceramint.2012.11.080.
Lin, D & Kwok, K W (2010) Structure and piezoelectric properties of new (Bi0.5Na0.5)1-x-yBax(Yb0.5Na0.5)yTiO3 lead-free ceramics. Journal of Materials Science: Materials in Electronics, 21(11), pp. 1119-1124. https://doi.org/10.1007/s10854-009-0029-7.
Li, D., et al. (2019) Structural evolution, dielectric and ferroelectric properties of (1-x) Bi0.5Na0.5TiO3-xBa0.3Sr0.7TiO3 ceramics. Journal of Materials Science: Materials in Electronics, 30(6), pp. 5917-5922. https://doi.org/10.1007/s10854-019-00890-4.
Chen, X., et al. (2014) Significant effects of powder preparation processes on the physical properties of Bi0.5Na0.5TiO3-0.06BaTiO3 ceramic. Journal of Materials Science: Materials in Electronics, 25(12), pp. 5309-5315. doi.org/10.1007/s10854-014-2306-3.
Guo, Y., et al. (2014) Structure, ferroelectric and piezoelectric properties of Bi0.5(Na0.8K0.2)0.5TiO3 modified BiFeO3-BaTiO3 lead-free piezoelectric ceramics. Journal of Materials Science: Materials in Electronics, 25(9), pp. 3753-3761. doi.org/10.1007/s10854-014-2086-9.
Barick, B. K., et al. (2013) Dielectric and impedance spectroscopy of zirconium modified (Na0.5Bi0.5)TiO3 ceramics. Ceramics International, 39(5), pp. 5695-5704. doi.org/10.1016/j.ceramint.2012.12.087.
Behara, S., et al. (2018) Structural, optical, and Raman studies of Gd doped sodium bismuth titanate. Ceramics International, 44(11), pp. 12118-12124. doi.org/10.1016/j.ceramint.2018.03.233.
Ruth, D. E. J., et al. (2016) Structural and electrical properties of bismuth magnesium titanate substituted lead-free sodium bismuth titanate ceramics. Journal of Materials Science: Materials in Electronics, 27(7), pp. 7018-7023. https://doi.org/10.1007/s10854-016-4658-3.
Hiruma, Y., et al. (2009) Thermal depoling process and piezoelectric properties of bismuth sodium titanate ceramics. Journal of Applied Physics, 105(8), p. 084112. doi.org/10.1063/1.3115409.
Hajra, S., et al. (2017) Structural and electrical characteristics of barium modified bismuth-sodium titanate (Bi0.49Na0.49Ba0.02)TiO3. Journal of Materials Science: Materials in Electronics, 29(2), pp. 1463-1472. doi.org/10.1007/s10854-017-8054-4.
Wang, J., et al. (2018) Dual relaxation behaviors and large electrostrictive properties of Bi0.5Na0.5TiO3-Sr0.85Bi0.1TiO3 ceramics. Journal of Materials Science, 53(6), pp. 8844-8854. doi.org/10.1007/s10853-018-2186-7.
Du, H., et al. (2007) Phase structure, microstructure, and electrical properties of bismuth modified potassium-sodium niobium lead-free ceramics. Journal of Applied Physics, 102(5), p. 054102. doi.org/10.1063/1.2775997.
Babu, M. V. G., et al. (2015) Enhanced piezoelectric constant and remnant polarisation in K-compensated sodium potassium bismuth titanate. Materials Letters, 146, pp. 81-83. doi.org/10.1016/j.matlet.2015.01.152.
Hiruma, Y., et al. (2007) Investigation of phase transition temperatures on (Bi1/2Na1/2)TiO3-(Bi1/2K1/2)TiO3 and (Bi1/2Na1/2)TiO3-BaTiO3 lead-free piezoelectric ceramics by electrical measurements. Ferroelectrics, 346(1), pp. 114-119. doi.org/10.1080/00150190601180471.
Li, S., et al. (2020) Piezoelectricity and flexoelectricity of sodium bismuth titanate-based ceramics. Ceramics International, 46, pp. 2049-2054.
Sahoo, S., et al. (2018) Resistive, capacitive and conducting properties of Bi0.5Na0.5TiO3-BaTiO3 solid solution. Ceramics International, 44(5), pp. 4719-4726. doi.org/10.1016/j.ceramint.2017.12.054.
Yang, M., et al. (2017) Doping effect of Ta5+ ions on microstructure and electrical properties of BaTiO3-(Bi0.5Na0.5)TiO3 ceramics with positive temperature coefficient of resistivity. Journal of Materials Science: Materials in Electronics, 28(14), pp. 10589-10595. doi.org/10.1007/s10854-017-6833-6.
Wang, J., et al. (2019) Intrinsic and extrinsic electric response in Bi0.5Na0.5TiO3-BaTiO3 lead-free piezoceramics. Ferroelectrics, 547(1), pp. 156-163. https://doi.org/10.1080/00150193.2019.1592495.
Cernea, M., et al. (2012) Structural, optical, and electric properties of BNT-BT0.08 thin films processed by sol-gel technique. Journal of Materials Science, 47(19), pp. 6966-6971. doi.org/10.1007/s10853-012-6646-1.
Bajpai, P. K., et al. (2016) Relaxor behavior and dielectric relaxation in lead-free solid solutions of (1-x) (Bi0.5Na0.5TiO3)-x(SrNb2O6). Journal of Electronic Materials, 45, pp. 928-939. https://doi.org/10.1007/s11664-015-4226-2.
Anem, S., et al. (2016) Investigation of lanthanum substitution in lead-free BNBT ceramics for transducer applications. Ceramics International, 42(14), pp. 15319-15326. doi.org/10.1016/j.ceramint.2016.06.173.
Sumang, R., et al. (2017) Synthesis and electrical properties of BNT-BKT-KNN lead free piezoelectric solid solution prepared via the combustion technique. Ferroelectrics, 518(1), pp. 11-22. doi.org/10.1080/00150193.2017.1360116.
Lu, Y Q & Li, Y X (2011) A review on lead-free piezoelectric ceramics studies in China. Journal of Advanced Dielectrics, 1(03), pp. 269-288. doi.org/10.1142/S2010135X11000409.
Sumang, R., et al. (2019) Phase transition and electrical properties of [(0.935-x)BNT0.065BT-xBZT] lead-free piezoelectric ceramics. Ferroelectrics, 552(1), pp. 148-158. doi.org/10.1080/00150193.2019.1653091.
Moosavi, A., et al. (2015) Effects of nano-sized BiFeO3 addition on the properties of high piezoelectric response (1-x)Bi0.5Na0.5TiO3-Bi0.5K0.5TiO3 ceramics. Journal of Materials Science, 50(5), pp. 2093-2102. doi.org/10.1007/s10853-014-8771-5.
Zhou, X., et al. (2020) High piezoelectric response and excellent fatigue resistance in Rb-substituted BNT-BKT-BT ceramics. Journal of Materials Science, 55(8), pp. 7634-7644. doi.org/10.1007/s10853-020-04571-0.
Trelcat, J. F., et al. (2012) Morphotropic phase boundary in the BNT-BT-BKT system. Ceramics International, 38(4), pp. 2823-2827. doi.org/10.1016/j.ceramint.2011.11.053.
Xiao, M., et al. (2018) Microstructure and dielectric properties of BaTiO3-(Bi0.5Na0.5)TiO3-NiNb2O6 ceramics. Journal of Materials Science: Materials in Electronics, 29(6), pp. 17689-17694. doi.org/10.1007/s10854-018-9874-6.
Mohanty, S. K., et al. (2020) Synthesis and dielectric spectroscopic study of lead-free ferroelectric ceramic K0.5Bi0.5TiO3-NaNbO3. Journal of Materials Science: Materials in Electronics, 31(4), pp. 3245-3255. https://doi.org/10.1007/s10854-020-02873-2.
Kang, W., et al. (2019) Enhanced dielectric and piezoelectric performance of sol-gel derived (1-x)Bi0.5(Na0.78K0.22)0.5TiO3-xBaTiO3 ceramics. Ceramics International, 45(17), pp. 23078-23083. doi.org/10.1016/j.ceramint.2019.07.356.
Halim, N. A., et al. (2016) Pyroelectric, ferroelectric, piezoelectric and dielectric properties of Na0.5Bi0.5TiO3 ceramic prepared by sol-gel method. Ceramics International, 42(14), pp. 15664-15670. doi.org/10.1016/j.ceramint.2016.07.022.
Jaiban, P., et al. (2013) High-and low-field dielectric responses and ferroelectric properties of (Bi0.5Na0.5) Zr1-xTixO3 ceramics. Ceramics International, 39, S81-S85. doi.org/10.1016/j.ceramint.2012.10.039.
Hong, C. H., et al. (2016) Lead-free piezoceramics-where to move on? Journal of Materiomics, 2(1), pp. 1-24. doi.org/10.1016/j.jmat.2015.12.002.
Zhang, X., et al. (2017) Phase transition and huge field-induced strain of BaZrO3 modified (Bi0.5Na0.5)0.94Ba0.06TiO3 ceramics. Journal of Materials Science: Materials in Electronics, 28, pp. 14664-14671. doi.org/10.1007/s10854-017-7331-6.
Cheng, R., et al. (2016) Giant piezoelectricity and ultrahigh strain response in bismuth sodium titanate lead-free ceramics. Materials Letters, 165, pp. 143-146. doi.org/10.1016/j.matlet.2015.11.131.
Taghaddos, E., et al. (2018) Fabrication and characterization of single-aperture 3.5-MHz BNTbased ultrasonic transducer for therapeutic application. IEEE Transactions on Ultrasonics, Ferroelectrics, and Frequency Control, 65(4), pp. 582-588. doi.org/10.1109/TUFFC.2018.2793874.
Taghaddos, E., et al. (2015) Lead-free piezoelectric materials and ultrasonic transducers for medical imaging. Journal of Advanced Dielectrics, 5(2), p. 1530002. doi.org/10.1142/S2010135X15300029.
Cernea, M., et al. (2018) Synthesis and characterization of novel ferrite-piezoelectric multiferroic core-shell-type structure. Journal of Materials Science, 53(13), pp. 9650-9661. doi.org/10.1007/s10853-018-2264-x.
Jaita, P., et al. (2018) The mechanical and electrical properties of modified-BNKT lead-free ceramics. Integrated Ferroelectrics: An International Journal, 187(1), pp. 147-155. doi.org/10.1080/10584587.2018.1444888.
Kantha, P & Pisitpipathsin, N (2018) Effect of KNbO3 addition on diffuse phase transition and dielectric properties of Bi0.5Na0.5TiO3 ceramics. Integrated Ferroelectrics: An International Journal, 187(1), pp. 129-137. doi.org/10.1080/10584587.2018.1444884.
Fernandez-Benavides, D. A., et al. (2018) Comparative study of ferroelectric and piezoelectric properties of BNT-BKT-BT ceramics near the phase transition zone. Materials, 11(3), p. 361. doi.org/10.3390/ma11030361.
Jeon, Y. H., et al. (2013) Dielectric and ferroelectric properties of (Bi0.5Na0.5) TiO3-(Bi0.5K0.5) TiO3-BaTiO3 thin films deposited via chemical solution deposition. Materials Letters, 106, pp. 63-66. doi.org/10.1016/j.matlet.2013.04.096.
Christensen, M., et al. (2017) Fabrication of lead-free Bi0.5Na0.5TiO3 thin films by aqueous chemical solution deposition. Materials, 10(3), p. 213. doi.org/10.3390/ma10020213.
Li, J., et al. (2020) Enhanced energy density in poly(vinylidene fluoride) nanocomposites with dopamine modified BNT nanoparticles. Journal of Materials Science, 55(6), pp. 2503-2515. doi.org/10.1007/s10853-019-04132-0.
Jeong, C K (2020) Toward bioimplantable and biocompatible flexible energy harvesters using piezoelectric ceramic materials. MRS Communications, 10(3), pp. 365-378. doi.org/10.1557/mrc.2020.48.
Zhang, Y., et al. (2020) Progress in lead-free piezoelectric nanofiller materials and related composite nanogenerator devices. Nanoscale Advances, 2(8), pp. 3131-3149. doi.org/10.1039/C9NA00809H.
Nanda, D., et al. (2019) Structural, dielectric, ferroelectric and magnetic properties of (BNT-BT)-NCZF composites synthesized by a microwave-assisted solid-state reaction route. Journal of Electronic Materials, 48(8), pp. 5039-5047. doi.org/10.1007/s11664-019-07304-5.
Yao, M., et al. (2016) Enhanced energy storage properties of (1-x) Bi0.5Na0.5TiO3-xBa0.85Ca0.15Ti0.9Zr0.1O3 ceramics. Materials Letters, 174, pp. 110-113. doi.org/10.1016/j.matlet.2016.03.089.
Pu, Y., et al. (2016) Phase transition behavior, dielectric and ferroelectric properties of (1-x)(Bi0.5Na0.5)TiO3-xBa0.85Ca0.15Ti0.9Zr0.1O3 ceramics. Journal of the European Ceramic Society, 36(10), pp. 2461-2468. doi.org/10.1016/j.jeurceramsoc.2016.03.005.
Fan, P., et al. (2016) Constrained sintering and electrical properties of BNT-BKT lead-free piezoceramic thick films. Ceramics International, 42(2), pp. 2534-2541. doi.org/10.1016/j.ceramint.2015.10.055.
Aoyagi, R., et al. (2005) Ferroelectric and piezoelectric properties of bismuth layered-structure ferroelectric (Sr, Na, Bi) Bi2Ta2O9 ceramics. Materials Science and Engineering: B, 116(2), pp. 156-160. doi.org/10.1016/j.mseb.2004.09.025.
Badapanda, T., et al. (2017) Dielectric, ferroelectric and piezoelectric study of BNT-BT solid solutions around the MPB region. IOP Conference Series: Materials Science and Engineering, 178(1), p. 012032. doi.org/10.1088/1757-899X/178/1/012032.
Smolensky G A (1961) New ferroelectrics of complex composition. IV. Materials Science, 2, pp. 2651-2654.