This article considers the quantization of bilayer graphene, based on research published in the Journal of Magnetism and Magnetic Materials.
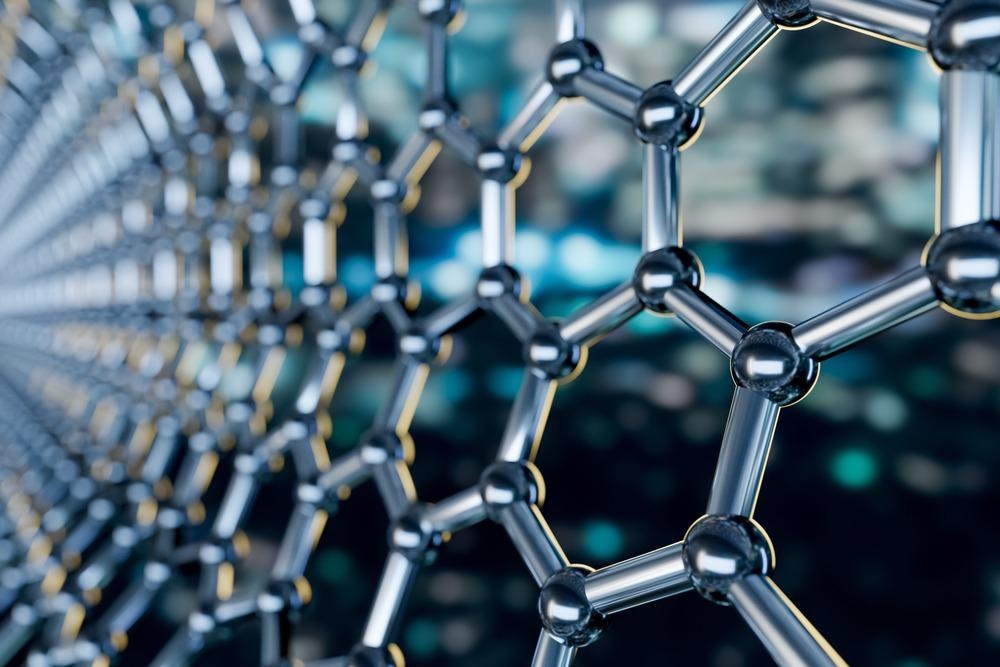
Image Credit: Production Perig/Shutterstock.com
Under certain conditions, a substance's electrical resistance can be 'quantized,' or assigned a set value that is irrespective of the material's basic characteristics.
This 'quantization' happens when electrons travel in two dimensions under high magnetic fields at very low temperatures. The electrical resistance is quantized under certain conditions, which means it varies in discrete stages rather than constantly. When electrons flow in a two-dimensional form under high magnetic fields and happen at very low temperatures, this quantization of electrical resistance happens.
The researcher at the University of Göttingen found the quantum Hall effect, QAH, or the quantization that happened in bilayer graphene. Standard microfabrication methods are used to contact the fragile graphene flakes, and the graphene flake is arranged such that the flake hangs naturally like a bridge, supported at the edges by two metal contacts.
At low temperatures and with nearly imperceptible magnetic fields, the highly pure double-layers of graphene reveal a quantization of electrical resistance. Furthermore, the electrical current can flow without wasting any energy. The explanation for this is a type of magnetism that is created by the mobility of charged particles in the graphene double-layer directly, rather than the normal approach as observed in conventional magnets.
This phenomenon is unique not only because it requires just an electric field, but also because it happens in eight different variations that may be controlled using an electric and magnetic field.
Since the effect can be turned on and off, and the direction of travel of the charged particles may be reversed, this leads to a high level of control. Furthermore, the fact that we can demonstrate this action in a system made up of a simple and found natural substance is a plus. This is in sharp contrast to the recently popularized 'heterostructures,' which need a complicated and exact mix of materials.
In the absence of an external magnetic field, the Quantum Anomalous Hall (QAH) isolator is a form of matter in which spontaneous violation of dynamic time symmetry causes the Quantum Hall effect. The QAH condition, first theorized in 1981, had not been confirmed until early 2021 by University of Göttingen researchers.
The shiftiness of the QAH state has been attributed to fluctuation in the new literature on contract-driven thin films, which often favor the QAH state over a Quantum Spin Hall (QSH) state, which in itself is degenerated only with the QAH state on the mean-field level.
This is important because double-layer graphene makes it a particularly appealing option for possible applications, such as the creation of novel computer parts in the field of Spintronics, which might have significance for high data storage.
One of the growing topics for the next nanoelectronics materials is Spintronics, which aims to lower power consumption while increasing memory and processing capacities. The electron's spins degree of freedom, which can react with its orbital energies, is used in such devices.
The spin polarization in these devices is regulated by magnetic layers acting as spin-polarizers or analyzers or by spin-orbit coupling interactions. Spin current can also be carried by spin waves. Spintronics is important because high-speed, reduced threshold current, high-power lasers, high-density logic, electronic memory devices, low power, and optoelectronic devices are all available from Spintronics. This technique is a massive source of circularly polarized light.
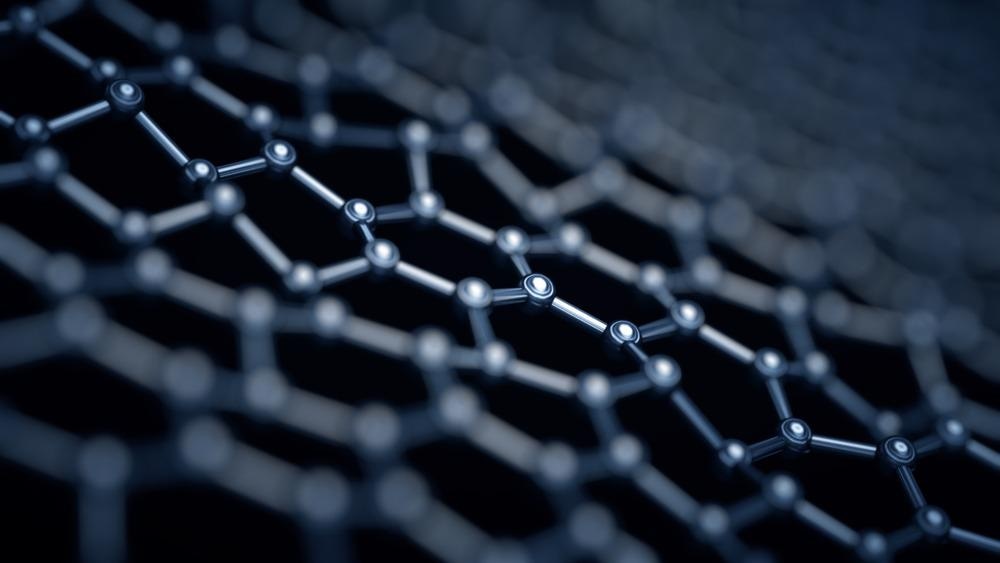
Image Credit: Egorov Artem/Shutterstock.com
The limitation of this new finding is how to stabilize the materials under high temperatures since the quantum only happens at temperatures up to five degrees over absolute zero at the moment.
The new method reveals that at low temperatures and with nearly imperceptible magnetic fields, the highly pure double-layers of graphene reveal a quantization of electrical resistance. This event requires not just an electric field but also because it happens in eight different variations and can turn it on and off.
The process is done by contacting graphene flakes using standard microfabrication procedures, and the flake is positioned such that it swings freely with two metal contacts supporting the flake at its edges. Meanwhile, the results show that the gapped state found in charge-neutral bilayer graphene in quantizing magnetic fields continues down to the low fields, eventually passing onto another gapped state at zero magnetic fields.
Further Reading: The Use of Graphene in Dentistry
The nature of the gapped state at zero fields, on the other hand, remains uncertain. As a result, elucidating the relationship between distinct gapped states and comprehending their physical features is a fun and timely endeavor.
This new finding suggests that there is an eight-fold degenerated Landau level at zero energy that contains both electrons and holes at the same time. Taking advantage of the narrow energy gap, bilayer graphene (or BLG) may be utilized to make field-effect semiconductors or tunneling field-effect transistors.
Because its bandgap can be modified by modifying the stacking orders and also generating an external electric field, BLG has a lot of promise as a novel material for optoelectronic devices. An imaging approach that can view and describe distinct stacking domains in BLG might be very useful in maximizing the benefits of BLG's features.
The limitation is that the process happens at low temperatures. The next research should see if the BLG can be quantized at high temperatures.
References
Hirohata, A., et al. (2020). Review on spintronics: Principles and device applications. Journal of Magnetism and Magnetic Materials. https://www.sciencedirect.com/science/article/pii/S0304885320302353
Geisenhof et al. (2021) Quantum anomalous Hall octet driven by orbital magnetism in bilayer graphene. Nature, 2021 DOI: 10.1038/s41586-021-03849-w
Nandkishore, R. and Leonid, L. (2010) Quantum Anomalous Hall State in Bilayer Graphene. Physical review. B, Condensed matter 82(11) DOI:10.1103/PhysRevB.82.115124
Disclaimer: The views expressed here are those of the author expressed in their private capacity and do not necessarily represent the views of AZoM.com Limited T/A AZoNetwork the owner and operator of this website. This disclaimer forms part of the Terms and conditions of use of this website.