There has been significant research into the practical uses of tin oxide (SnO2), particularly in applications such as transparent electrodes, electromagnetic shielding, n-type thin-film transistor (TFT) solar cells and gas sensors.
Most of the benefits associated with tin oxide are related to its exceptional semiconductor properties, low resistance and high optical transparency in the visible range.
Despite its broad applicability and valuable characteristics, there have been relatively few studies focused on the preparation of SnO2 films using high-power pulsed magnetron sputtering.
High-power pulsed magnetron sputtering (HiPIMS) technology is based on conventional magnetron sputtering technologies. This technique has attracted significant attention due to its exceptionally high target ionization rate.
At its core, HiPIMS works by increasing the peak power of the pulses, allowing a magnetron sputtering technique to deliver a high sputtering metal ionization rate while ensuring a low pulse duty cycle.
The application of power in pulses with a high amplitude and a low duty cycle allows the ionization of large fractions of sputtered atoms and near-target gases. The dense plasma in front of the target helps maintain the discharge while simultaneously assisting film growth by modifying the growing films’ microstructure.
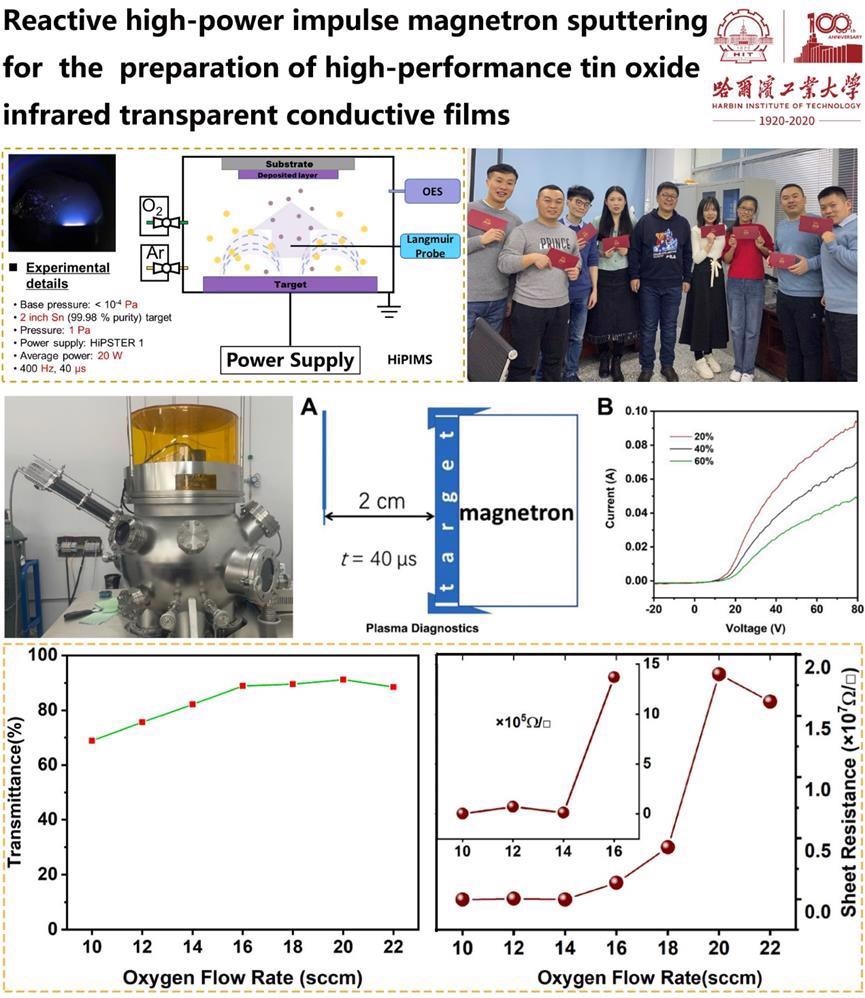
Image Credit: Hiden Analytical
Oxygen content is an essential parameter in the fabrication of SnO2 thin films via high-power pulsed magnetron sputtering. It is important to note that the film’s oxygen ratio may differ from the oxygen partial pressure rate provided by the flowmeter.
Oxygen vacancies will result in lattice distortion, impacting the mobility of charge carriers in the SnO2 film, highlighting just how much oxygen partial pressure during the sputtering deposition process affects the SnO2 films’ conductivity.
A research team led by Professor Jiaqi Zhu from the Harbin Institute of Technology (HIT) conducted a study into the reaction mode of high-power pulsed reactive magnetron sputtering.
The study also sought to explore the crystal structure and infrared transparent conductive properties of SnOx thin films that had been prepared at 600 °C.
The research focused on the effects of sputtering at various oxygen partial pressures from 10 to 24 sccm.
It was noted that the reaction mode was dominated by the metallic mode for SnOx films deposited at oxygen partial pressures of 10–14 sccm. It was also determined that the polar unsaturated (101) plane was the favored orientation of the film crystals.
The reaction mode was dominated by the transition mode for SnOx films deposited at oxygen partial pressures of 16–18 sccm. These exhibited a preferred (110) plane orientation. In the deposition process, the reaction proceeded in poisoning mode at oxygen partial pressure of >18 sccm.
One key finding of the study was that as oxygen partial pressure increased, the carrier concentration reduced to 1.140 × 1015 cm-3, IR transmittance at 4 μm increased, and mobility increased to 14.93 cm2/Vs.
Excess O2 also reduced the electrical properties of the prepared SnOx films, offering a maximum resistivity of 502.9 Ω·cm.
The researchers utilized a commercial electrostatic Langmuir probe (ESPion, Hiden) to observe the plasma’s state at various stages of the process. The measured probe current as a function of the voltage was applied to the probe at different typical oxygen partial voltages.
The team also included Liangge Xu, a Ph.D. student of HIT. Dr. Lei Yang, the Department of Center of Analysis Measurement of HIT.
Reference
“Reaction mode-controlled crystal structure and optical and electrical properties of SnOx infrared transparent conducting films” Surface and Coatings Technology (2021) 422, 127506 https://doi.org/10.1016/j.surfcoat.2021.127506
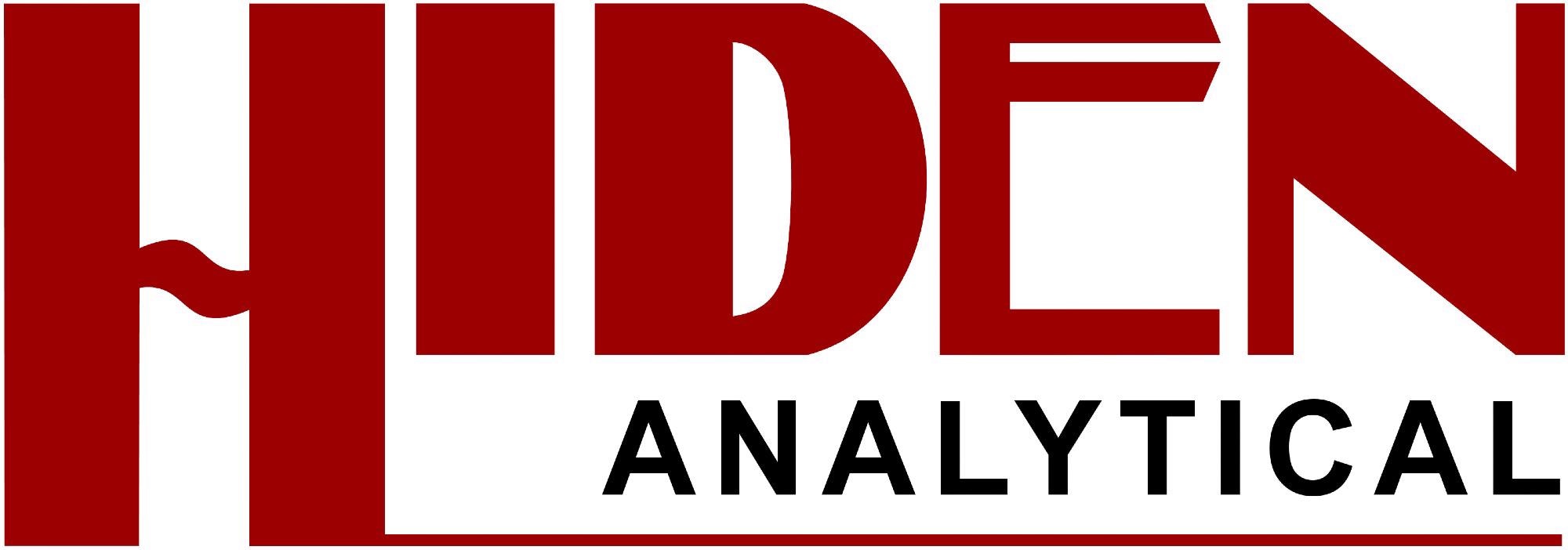
This information has been sourced, reviewed and adapted from materials provided by Hiden Analytical.
For more information on this source, please visit Hiden Analytical.