The high sensitivity of the reflection electron microscopy (REM) technique to small changes in the crystal structure and composition of the top surface layers of various crystalline materials makes it the method of choice when studying surface structures and dynamic surface processes.
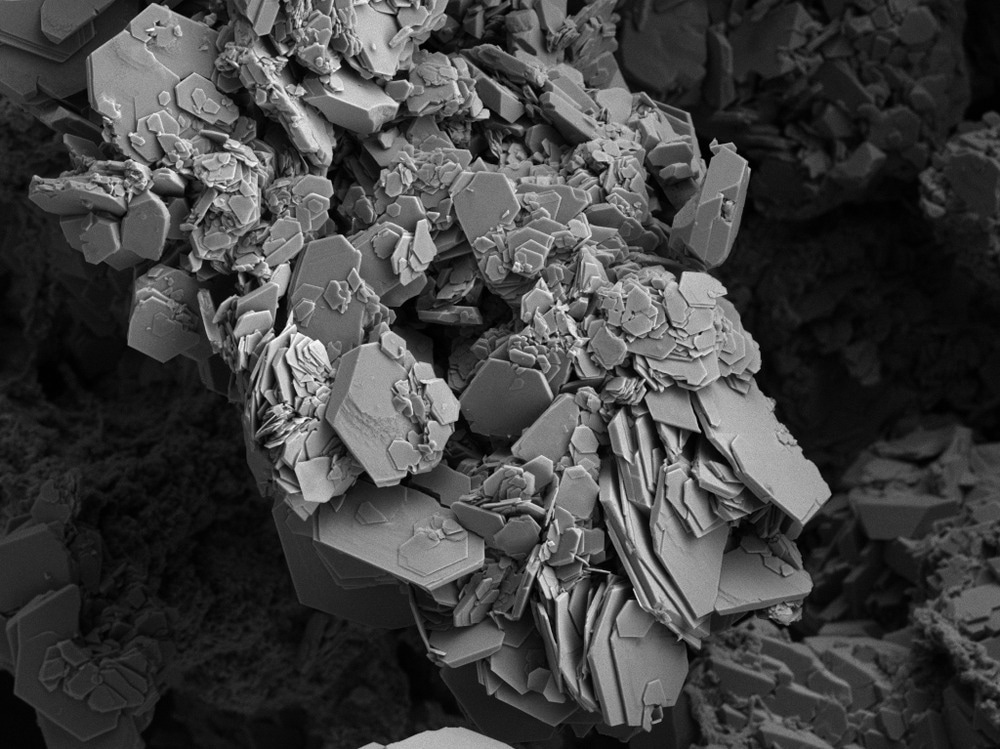
Image Credit: Ravenash/Shutterstock.com
Numerous diffraction, spectroscopic, and microscopy techniques are suitable for the characterization of thin films and surfaces, but only the electron microscopy-based methods can provide direct real-space information about surface defects and inhomogeneities with a sub-nanometer resolution.
Surface Characterization with High Spatial Resolution
To surpass the resolution limit of optical microscopy when imaging surface topography, Nobel laureate Ernst Ruska developed the reflection electron imaging technique shortly after the invention of transmission electron microscopy (TEM) in 1933. Reflection electron microscopy (REM) uses very similar instrumentation to TEM, with the main difference being that the high-energy electron beam (100–200 kV acceleration voltage) interacts with the sample surface at a glancing angle of incidence (5-10 degrees), thus the sample therefore no longer needs to be thin and electron-transparent.
Later on, the technique was further improved by employing the reflection high-energy electron diffraction (RHEED) of a Bragg-reflected electron beam to form high-resolution and high-contrast REM images. The reflected electrons from the sample surface form a diffraction pattern at the back-focal plane of the electron microscope.
By selecting an appropriate diffraction peak using the objective aperture, the researchers can obtain images that are highly sensitive to small changes in the crystal structure, orientation, or composition of the few topmost atomic layers of the sample surface. Such information cannot be obtained using alternative electron microscopy techniques, such as scanning electron microscopy and secondary electron imaging, and is of great value in surface science.
REM permits scientists to combine imaging, diffraction, and spectroscopy approaches for the characterization of the surface topography, crystal structure, and composition of crystals.
Drawbacks of Using Grazing Incidence Electron Beams
However, employing a glancing electron beam, which enables the superior surface sensitivity of the technique, causes a severe foreshortening of the obtained images. As a result, the magnification is roughly 50 times higher in the direction perpendicular than in the direction parallel to the electron beam. This effect severely limits the image resolution in the direction of the incident electron, limiting the high-resolution data to be obtained only across the sample surface, perpendicular to the beam.
Correction of the image foreshortening is of great importance for the quantitative characterization of the sample surface topology. Over the past three decades, the improvements in electron optics, detector technology, and numerical methods for image correction enabled the development of a low-distortion REM technique, where the image foreshortening is greatly reduced.
Despite this drawback, the scientific value of the technique is greatly appreciated by scientists and engineers, as it enables the visualization of single-atomic steps on crystal surfaces.
Imaging Steps on Crystal Surfaces
Because of the glancing angle of incidence, the amount of defocus occurring in a REM image can be very large (several micrometers), resulting in a strong contrast over the entire field of view. Analyzing the phase contrast in a through-focus series of images can provide a straightforward way of determining the direction of the steps on the crystal surface (up-step or down-step features).
Image Contrast Arising from Dislocations and Surface Faults
Because the contrast in a REM image derives from the variation of intensity of a diffracted beam, the small changes in the crystal lattice structure and orientation can be visualized. For example, when a dislocation emerges from the bulk and intersects the crystal surface, the associated strain fields distort the surface, resulting in a characteristic striation extending across the surface (perpendicular to the incident beam), which is easily observable in the REM image. The type of dislocation can be derived from the configuration of the striation pattern and its dependence on the diffraction conditions.
The sensitivity to the variations in the lattice strain is so high that REM images show a strong contrast between surface regions with a minimal structural difference. Besides, small surface defects and impurities are easily detected, making the technique highly suitable for real-time monitoring of in situ surface treatments, structural phase transitions, adsorption processes, crystal growth or evaporation, and nanostructure formation.
Applications of Quantitative REM Imaging
REM images can be correlated with RHEED, reflection electron energy loss, and energy-dispersive X-ray spectroscopy data to determine the surface chemical composition with nanometer spatial resolution. The capability of REM to image dynamic surface features makes the technique suitable for the semiconductor industry to observe processes, such as epitaxial crystal growth, dopant migration on semiconductor surfaces, and morphological changes in crystals under different conditions, including electric fields and variable temperatures.
An application with great potential is the REM-based electron holography, where the electron beam reflected from the area of interest on the sample surface interferes with a reference beam reflected from an adjacent perfectly flat area of the crystal. Scientists have demonstrated that using this approach, step heights or strain fields around emerging dislocations can be mapped with a resolution of less than 0.01 nm.
More from AZoM: Auger Electron Spectroscopy in the Semiconductor Industry
References and Further Reading
Lehmpfuhl, G., Uchida, Y. (1990) Observation of surface crystallography by reflection electron microscopy. Surface Science, 235, 295-306. Available at: https://doi.org/10.1016/0039-6028(90)90805-I
Yagi, K. (1993) Reflection electron microscopy: studies of surface structures and surface dynamic processes. Surface Science Reports, 17, 307-362. Available at: https://doi.org/10.1016/0167-5729(93)90002-7
Wang, Z. (1996) Reflection Electron Microscopy and Spectroscopy for Surface Analysis. Cambridge: Cambridge University Press. Available at: https://doi.org/10.1017/CBO9780511525254
Disclaimer: The views expressed here are those of the author expressed in their private capacity and do not necessarily represent the views of AZoM.com Limited T/A AZoNetwork the owner and operator of this website. This disclaimer forms part of the Terms and conditions of use of this website.