Sponsored by Xenocs SASReviewed by Louis CastelAug 10 2022
Being the predominant component of plant fiber, cellulose is the most abundant organic compound on Earth.1 As a result of its exceptional mechanical properties, biodegradability, natural abundance, renewability and low cost, cellulose is considered a promising environmentally-friendly substitute for conventional petroleum-based polymers with application potential across a wide range of fields such as hydrogels, aerogels, high tenacity rayon or transparent films.2
When manufacturing cellulose-based products, challenges, such as the high melting point of cellulose, make it impossible for it to be melted without decomposition.
Thus, shaping cellulose materials typically demands the dissolution of the cellulose polymer and subsequent regeneration. For example, regenerated cellulose fibers are dry jet wet spun from ionic liquid solutions.
It is thus crucial to understand any structural changes down to the nano-scale during the production process. In this context, wide-angle and small-angle X-Ray scattering (WAXS and SAXS) measurements are excellent probing techniques that have the capacity to reveal both the evolution of the microstructure and of the crystalline order.
Additionally, the mechanical properties of most cellulose-based products depend greatly on the micro-scale and nano-scale structure of the interface between cellulose surfaces and other materials, especially when still in the wet state as the joints develop and then consolidate during the drying process.4
Grazing incidence small angle X-Ray scattering (GISAXS) is a versatile tool for the characterization of nanoscopic objects at buried interfaces, surfaces, or thin films.
This article describes how SAXS and WAXS measurements have been used to:
- Reveal the structural variations that occur during the drying process of cellulose beads swollen with various water/ethanol mixtures and containing different cellulose concentrations,
- Establish the differences in the crystallinity of regenerated cellulose fibers generated using different draw ratios.
Nanostructural Evolution of Cellulose Beads During Drying Investigated by SAXS
Recently, a team of researchers from the Jülich Centre for Neutron Scattering and Biological Matter and KTH Royal Institute of Technology conducted a comprehensive study of the nanostructure evolution on a model system made up of regenerated cellulose beads.
The objective was to assess the influence of the cellulose concentration and the non-solvent type and concentration.2,3
The cellulose beads were manufactured by introducing a solution of cellulose/lithium chloride (LiCl)/N, N-dimethylacetamide (DMAc) into a non-solvent (ethanol or water).
Thereafter, time-resolved SAXS measurements conducted on both water and ethanol swollen beads were used to monitor the nano-scale structural changes that appeared during drying.
Due to the shape of the SAXS curves, three drying regimes can be indentified for the water-swollen beads, with only two regimes for the ethanol-swollen counterparts by comparison.
To quantitatively assess the structural changes that occur during the drying process, the data was evaluated using a Guinier-Porod model. Tracking the evolution of some of the fitting parameters as a function of drying time facilitates an enhanced understanding of the structural changes in each regime.
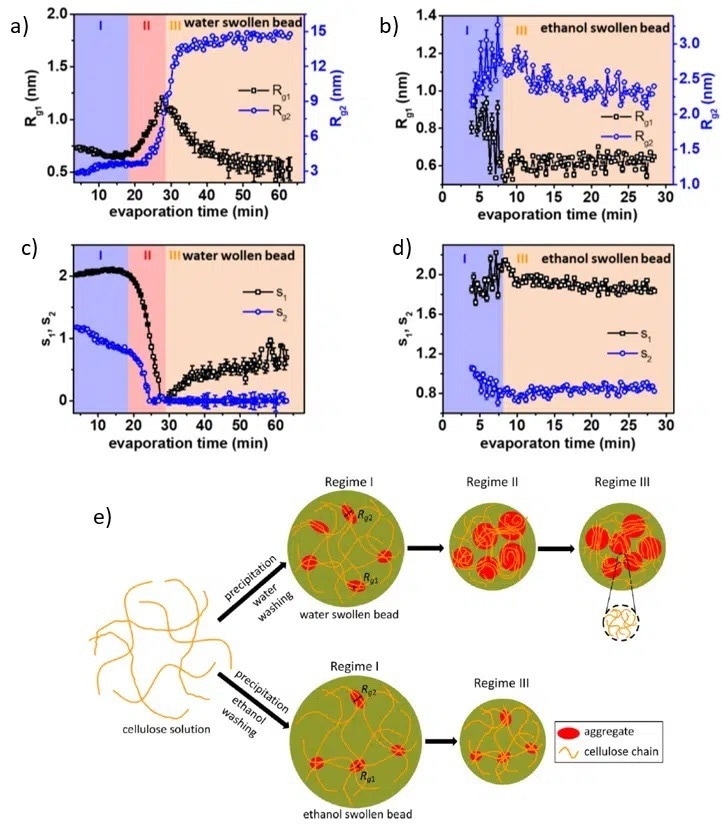
Figure 1 (a)-(d) Evolution of two characteristic length scales (Rg1 and Rg2) and two dimensionality parameters (s1 and s2) as a function of drying time and nonsolvent. (e) Graphical illustration of the structural evolution during nonsolvent evaporation. Image Credit: ACS Nano, 2020, DOI: 10.1021/acsnano.0c00171.
Knowing the fundamentals of cellulose structure and the order of magnitude, the two characteristic length scales, Rg1 and Rg2, can be associated with the size of the cellulose monomer and the size of an extended aggregate structure (see Figure 1 (e)).
The shape of the analogous scattering objects is determined by s1 and s2, the two dimensionality parameters:
- Spherical symmetry s1 = s2 = 0,
- Cylindrical objects s1 = 1, s2 = 0,
- Lamellae with equal width and length s1 = 2, s2 = 0. During the evaporation of the non-solvent, it is possible to describe the structural evolution by interpreting the values of all the parameters in each regime (as shown in Figures 1 (a)-(d)).
Water- Swollen Beads Evolution (Figures 1 (a) and (c))
Regime I (Evaporation time < 18 minutes)
Prior to the drying process commencing, the cellulose monomers exhibit a lamellar stacking structure of size in the order of 1 nm (corresponding to Rg1) in combination with larger extended structures with the shortest size being approximately 3 nm (related to Rg2).
Regime II (Evaporation time 18 – 28 minutes)
The accelerated decrease of both s1 and s2 to 0 signals the formation of structures with spherical symmetries. These larger structures form by merging already existing extended aggregates, as demonstrated by the increase in both Rg1 and Rg2.
Regime III (Evaporation time >28 minutes)
The objects with smaller characteristic sizes form rod-like structures with a size of around 0.5 nm, while the larger ones keep spherical symmetry.
Ethanol- Swollen Beads Evolution (Figures 1 (b) and (d))
Regime I and III
A structure similar to the water-swollen beads is seen in the swollen state (regime I). It is made of staked monomers and larger extended structures. Furthermore, no significant structural changes are observed during the evaporation process.
Crystalline Order Evolution of Cellulose Beads During Drying Investigated by WAXS
WAXS measurements were used in the investigation of whether crystalline order is formed as the evaporation process takes place.
For water-swollen beads, three peaks can be seen in the spectra shown in Figures 2 (a) and (b): the scattering peak from water (q = 20 nm-1) and two peaks associated with the (110) (q = 8.8 nm-1) and (110) (q = 14.6 nm-1) crystallographic planes of cellulose II structure.
The water peak shows a significant decrease in intensity as a function of evaporation time, as expected, while the cellulose peaks only appear at the start of regime II (18 minutes) and continually increase in intensity throughout the rest of the drying process.
A peak shift along the (110) direction is seen (Figure 2 (a)), suggesting that at the start of regime II, a cellulose II hydrate structure forms, accompanied by a continuous transition to cellulose II structure.
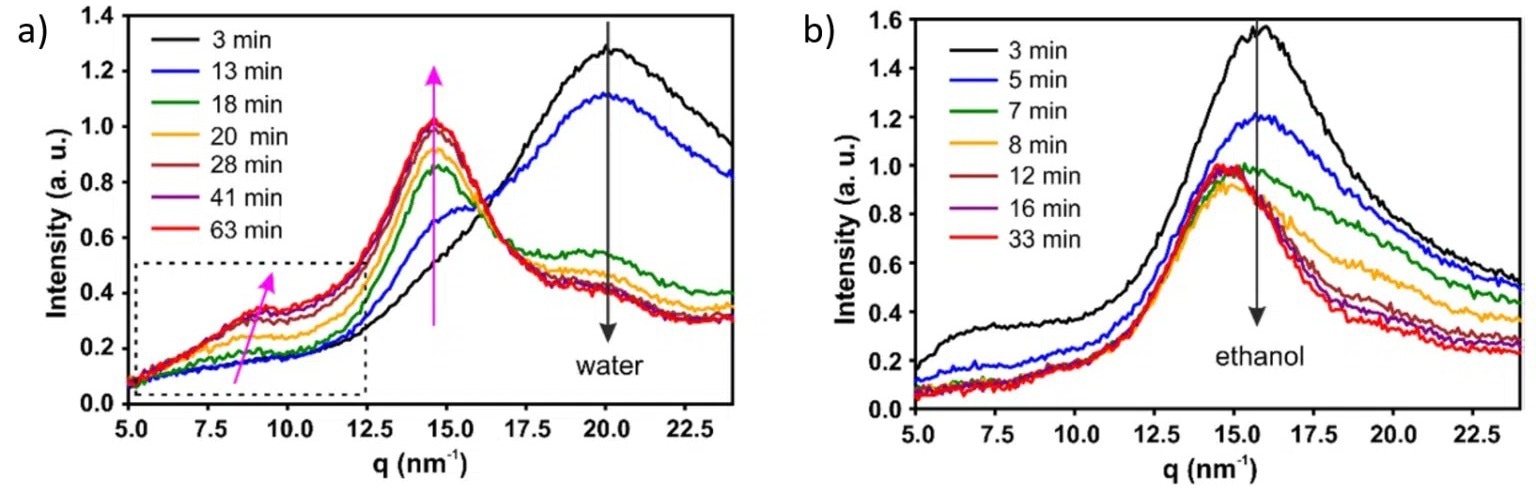
Figure 2. (a), (b) WAXS curves recorded at different times during drying process of water and ethanol swollen beads, respectively, with 1.5 wt% cellulose concentration. Image Credit: ACS Nano, 2020, DOI: 10.1021/acsnano.0c00171.
On the other hand, for ethanol swollen beads, no crystalline structures form during the drying process. In this instance, only a change in intensity of both the ethanol (q = 15.5 nm-1) and cellulose (q = 14.6 nm-1) peaks is seen during the evaporation of the non-solvent.
Furthermore, by conducting SAXS/WAXS experiments on cellulose samples swollen with various water/ethanol mixtures which contain varying cellulose concentrations, it was possible to develop a phase diagram (Figure 3) that facilitates easy prediction of the drying behavior of regenerated cellulose.
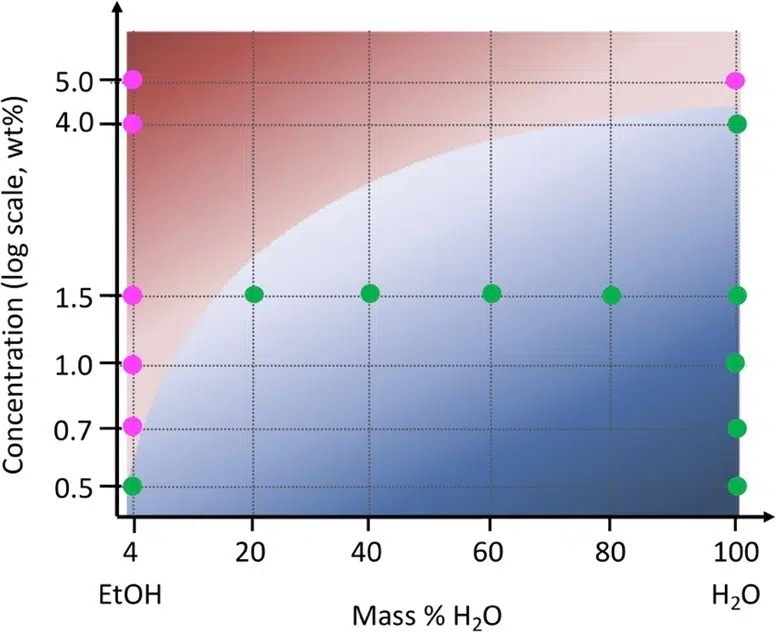
Figure 3. Phase diagram showing the structural evolution during drying of cellulose bead as a function of nonsolvent mixture. Magenta points correspond to a two-step drying process with no change in the cellulose structure, while green points represent a three-step drying process including a sharp structural change. Image Credit: ACS Nano, 2022, DOI: 10.1021/acsnano.1c09338.
The Crystallinity of Regenerated Cellulose Fibers
Recently, a study of the crystallinity of regenerated cellulose fibers was conducted by a group of researchers from Aalto University, Lund University, the Institute of Crystallography and the University of Bari.5
Generally, the crystallization of polymers is associated with only a partial alignment of molecular chains through cooling, mechanical stretching, or solvent evaporation. Therefore, the result is a combination of amorphous and crystalline domains, the ratio of which is tunable by processing parameters such as the draw ratio (DR).
The effect of the draw ratio on the crystallinity of regenerated cellulose fibers has been evaluated using wide-angle X-Ray scattering measurements.
To precisely determine the degree of fiber crystallinity (ϕc), such as the volume fraction of the crystalline domains, WAXS patterns were decomposed into amorphous and crystalline contributions as presented in the inset of Figure 4.
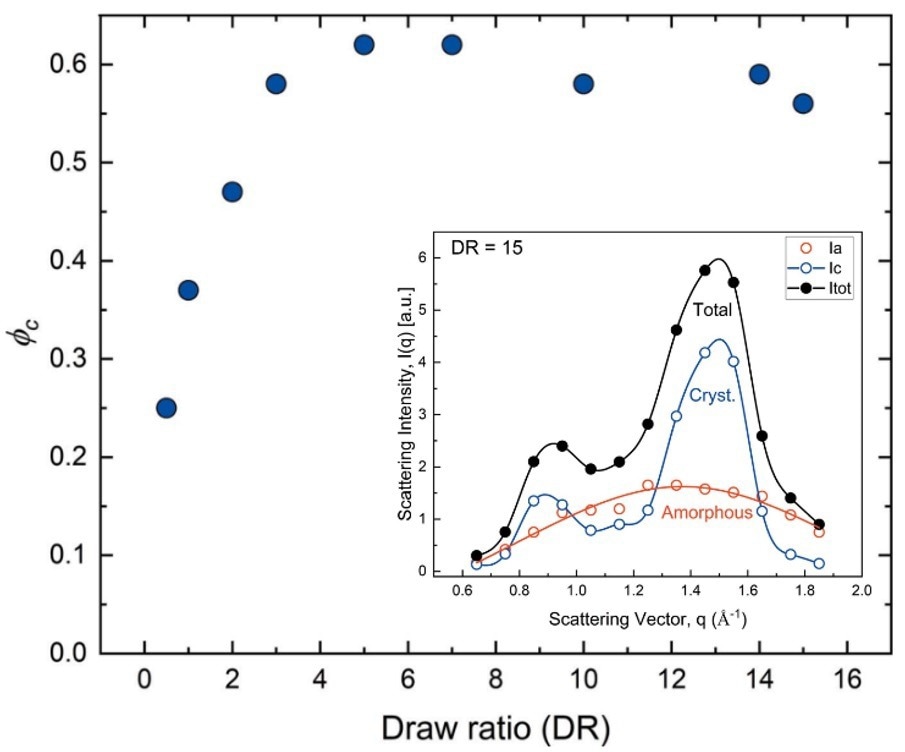
Figure 4. Fiber crystallinity obtained at different draw rations (DR). (inset) Individual contributions to the WAXS pattern of the crystalline and amorphous domains recorded on a DR 15 sample. Image Credit: IUCrJ, 2022, DOI: 10.1107/S205225252200570X.
As a function of increasing draw ratio, ϕc sharply increased up to DR = 5 and saturated to a value of ϕc = 0.6 ± 0.05 for all higher draw ratios. This behavior corresponds to the evolution of the mechanical properties of fibers generated using different DRs.
The tensile strength demonstrated an increase by a factor of 2 at low draw ratios (DR < 5), followed by a slower, continuous increase at higher draws.6
Conclusions
The drying kinetics of cellulose beads which contain a variety of cellulose concentrations and swollen in various non-solvent water/ethanol mixtures, have been assessed comprehensively with the application of SAXS and WAXS measurements.
These results show the different structural evolutions throughout the drying process depending on the cellulose concentration and the non-solvent used. Moreover, it was demonstrated that WAXS measurements are an exceptional tool to probe the crystallinity level of regenerated cellulose fibers created with different draw ratios.
This work offers invaluable information regarding the mechanisms that occur throughout the drying of regenerated cellulose and the influence of processing parameters. These insights are critical in the manufacturing process of various cellulose-based products.
Discover more applications of SAXS by downloading this SAXS Application Overview
References and Further Reading
- Wang, Sha, Tian Li, Chaoji Chen, Weiqing Kong, Shuze Zhu, Jiaqi Dai, Alfredo J. Diaz et al. “Transparent, anisotropic biofilm with aligned bacterial cellulose nanofibers.” Advanced Functional Materials 28, no. 24 (2018): 1707491.
- Li, Hailong, Margarita Kruteva, Martin Dulle, Zhen Wang, Katarzyna Mystek, Wenhai Ji, Torbjörn Pettersson, and Lars Wågberg. “Understanding the Drying Behavior of Regenerated Cellulose Gel Beads: The Effects of Concentration and Nonsolvents.” ACS nano 16, no. 2 (2022): 2608-2620.
- Li, Hailong, Margarita Kruteva, Katarzyna Mystek, Martin Dulle, Wenhai Ji, Torbjörn Pettersson, and Lars Wågberg. “Macro-and microstructural evolution during drying of regenerated cellulose beads.” ACS nano 14, no. 6 (2020): 6774-6784.
- Li, Hailong, Stephan V. Roth, Guillaume Freychet, Mikhail Zhernenkov, Nadia Asta, Lars Wågberg, and Torbjorn Pettersson. “Structure development of the interphase between drying cellulose materials revealed by in situ grazing-incidence small-angle X-ray scattering.” Biomacromolecules 22, no. 10 (2021): 4274-4283.
- Gentile, Luigi, Herbert Sixta, C. Giannini, and U. Olsson. “A novel X-ray diffraction approach to assess the crystallinity of regenerated cellulose fibers.” IUCrJ 9, no. 4 (2022).
- Asaadi, Shirin, Michael Hummel, Patrik Ahvenainen, Marta Gubitosi, Ulf Olsson, and Herbert Sixta. “Structural analysis of Ioncell-F fibres from birch wood.” Carbohydrate polymers 181 (2018): 893-901.
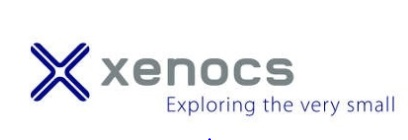
This information has been sourced, reviewed and adapted from materials provided by Xenocs.
For more information on this source, please visit Xenocs.