Hydraulic applications rely upon fluid power or pressurized fluids to generate, control, and transmit power. These systems are capable of producing high power and high forces in small volumes and have extended service lives.
Fluid power is utilized across diverse industries, including machine tools, self-propelled scissor lifts, steel production and metal extraction, paper and textile machinery, injection molding, and security and parking barriers, among others.
In these systems, pressure sensors play a crucial role, serving as essential components in a control loop with a Program Logic Controller (PLC) to regulate fluid pumps and maintain system pressure within a specified range.
The failure of the pressure sensor in such applications could result in an interruption in the feedback to the control of a motor, potentially causing equipment failure, costly downtime, or safety hazards for workers using the equipment.
Challenges and Top Five Specifications to Consider for Pressure Sensor Selection
Before selecting the appropriate pressure sensor for a system, it is crucial to understand the environment and operating conditions of the application. In hydraulic applications, tackling some of the typical challenges aids in identifying the most suitable specifications for the job.
High Pressure
These applications are distinguished by high fluid pressures that exceed 50 bar (725 psi), necessitating robust components that have been explicitly engineered to handle these elevated pressures to ensure reliable and safe operation.
In many of these systems, the fluids may be hazardous, and in combination with the high pressures and temperatures, the leak could become a hazard.
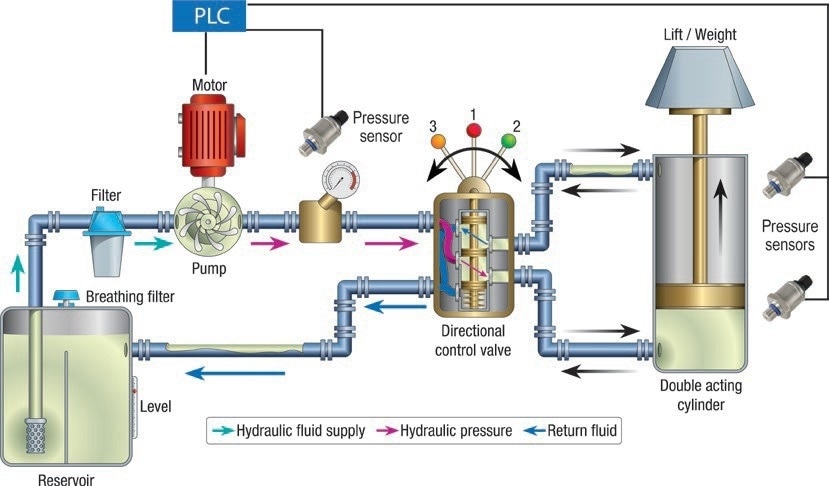
Figure 1. Pressure sensors play critical roles in hydraulic applications which typically involve high system pressures of greater than 50 bar (725 psi). Image Credit: Sensata Technologies, Inc.
Sense Element Technologies
There are a few sensor technologies frequently employed in pressure sensors for high-pressure range applications exceeding 50 bar (725 psi), each with its advantages and disadvantages.
Thick Film Ceramic sensing elements consist of four resistors arranged in a Wheatstone bridge configuration that are printed onto the non-process side of a ceramic substrate.
When pressure is exerted on the process side, the ceramic substrate undergoes deformation, leading to a physical alteration in the resistors and, consequently, an electrical output signal change.
In high-pressure systems such as hydraulic applications, the ceramic substrate is more vulnerable to cracking and breaking due to frequent high-pressure cycling and spikes than alternative metal substrate technologies.
The ceramic sense elements cannot be welded to the process connection, necessitating the use of an o-ring and locking mechanism to establish the seal. This increases the complexity of the sensor design and creates potential points of leaks and failures.
Thin Film Metal sensing elements employ the technique of thin film deposition to create a Wheatstone bridge pattern of resistors on the substrate. In this case, the functioning of the sensor’s electronics is akin to that of thick film ceramic.
Thin film technology has the advantage that the sputtering process can be easily applied to various types of materials. Additionally, as the sensing element is made of metal (often stainless steel), it can be welded into the process port design, forming a hermetic seal without requiring o-rings.
One potential issue with this type of sensor element design is that poor bonding between the thin film sensor element and the port material could lead to early component failure during endurance pressure cycling.
The thin film manufacturing process requires expensive sputtering equipment and a clean room, which can increase production costs.
Micro Silicon Strain Gauge sensing elements are made by bonding a silicon strain gauge to a metal substrate (typically 17-4 PH or 316 L stainless steel) using glass bonding technology.
The pressure port of the sensor is welded to the substrate, resulting in a hermetic process connection. This welding technique eliminates the need for an o-ring, thereby minimizing the risk of process fluid leakage into the sensor.
The small size of Micro Silicon Strain Gauge sensing elements offers greater design flexibility compared to other sensing element technologies.
The Micro Silicon Strain Gauge sensing elements exhibit exceptional glass bonding between the gauge and the stainless-steel port, resulting in a more robust technology than thin film alternatives when subjected to pressure cycling.
While thin film sensing elements may offer a more linear raw output, Micro Silicon Strain Gauge technology can achieve similar accuracy levels through calibration, resulting in a sensor that is more cost-effective while delivering the same or superior accuracy at the sensor level.
This technology has the advantage of higher sensitivity (mV/V) compared to a thin film, allowing for a thicker pressure-sensing element membrane, which in turn increases the burst strength.
High-Pressure Spikes and Burst Strength
Exceeding a component’s rated burst or proof pressure due to high-pressure spikes can result in component damage and failure.
Burst pressure is the maximum pressure a device can be exposed to for two minutes without rupture and is generally specified as a multiple of the upper limit of the device’s operating pressure range.
Even if repeated high-pressure spikes remain below a component’s rated proof and burst pressures, they can still cause damage to pressure diaphragms over time, potentially leading to sensor failure and system leak
The internal port geometry and diaphragm thickness of the sensing element are key factors contributing to a sensor’s burst pressure capacity. In addition, snubbers can be employed to safeguard the pressure sensor against repeated high-pressure spikes.
Snubbers function by decreasing the size of the aperture through which the fluid flows, attenuating the pressure spike before it reaches the sensing element. This is particularly useful in hydraulic systems utilized in construction machinery, where sudden stops or starts, or rapid turns of the vehicle, are common.
In such scenarios, the hydraulic system and its components are exposed to substantial pressure spikes, making a 10x burst strength rating a vital requirement to guarantee the integrity of the structure and operational continuity of the system.
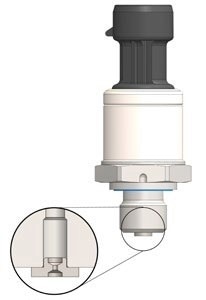
Figure 2. Pressure sensors like Sensata’s PTE7100 have greater than or equal to 10x burst strength up to full scale pressure range of 600 bar and can be configured with a snubber to dampen pressure pulses and help prevent component failure in hydraulic applications where high-pressure spikes are a concern. Image Credit: Sensata Technologies, Inc.
Shock and Vibration
Hydraulic systems typically consist of pumps, motors, and large cylinders, all of which are constantly moving and generating vibration. These systems are often integrated into larger systems that may be subjected to or cause higher levels of shock and vibration.
If a component is exposed to levels beyond what it was designed to withstand, it could result in damage to the mechanical construction and electronics. Heavy vehicle applications, such as off-road equipment, scissor lifts, or forklifts, are especially susceptible to shocks and higher levels of vibration.
Mechanical shock is a brief, momentary impact that can transmit a sudden burst of energy to a component due to transportation or rough handling. On the other hand, vibration is characterized as a continuous mechanical oscillation that can result from the operation of machinery such as engines or from an initial shock.
In hydraulic applications and mobile machinery, compressors, and engines that make up a system, pressure sensors are frequently subjected to mechanical shock and vibration.
To select an appropriate pressure sensor for use in such applications, it is crucial to evaluate the test standards that suppliers use to rate their sensors. This assessment ensures that the component is capable of withstanding the possible shock and vibration of the system.
To demonstrate the level of mechanical shock that their sensors can handle, pressure sensor suppliers frequently subject their sensors to IEC 60068-2-27 standards testing. A best-in-class rating of 500 g minimum, for instance, would prevent potential damage and component failure in hydraulic applications.
Moreover, in such systems, the continuous operation of compressors and motors means that all components are subjected to prolonged exposure to vibration.
Suppliers that offer more rigorous testing and vibration ratings for sensors subject their sensors to IEC 60068-2-6 standards testing. Qualifying a sensor to 30 g (10…2000 Hz) would result in more dependable and durable sensing solutions for the application.
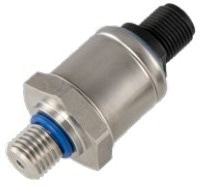
Figure 3. Pressure sensors like Sensata’s PTE7100 is rated to 30 g (10…2000Hz) vibration and 500 g min mechanical shock making it a robust and reliable solution for hydraulic applications where components are frequently exposed to harsh conditions. Image Credit: Sensata Technologies, Inc.
Electromagnetic Compatibility (EMC)
Due to the high power levels and intricate machinery frequently utilized in hydraulic applications, the equipment is susceptible to electromagnetic emissions and electrostatic discharges that could potentially harm the system if its components are not rated appropriately.
For instance, a hydraulic pump without a proper ground connection emits electrical noise or interference into the surrounding environment. Another example could be an electric welding machine being used on a mobile aerial work platform without a solid ground connection.
In all of these examples, there is a heightened risk of electromagnetic emissions that could potentially disrupt the signal of the sensor.
In such scenarios, selecting sensors with strict EMC standards, including Enhanced Radiated Immunity (EMI) ratings of at least 150 V+/m and enhanced Electrostatic Discharge (ESD) ratings of ±8 kV contact or ±15 kV air, will help to ensure sufficient protection.
Pressure Sensors and Their Critical Functions in Industrial Applications
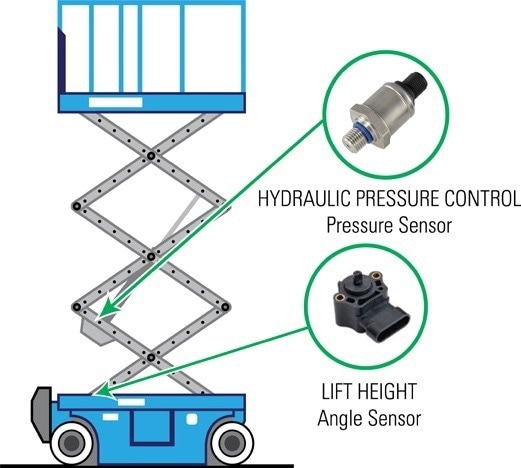
Figure 4. Pressure sensors play critical roles in scissor lifts, where they control the hydraulic pressure of the main cylinder or, when combined with an angle sensor, they can help control platform overload conditions. Image Credit: Sensata Technologies, Inc.
Safety in Scissor Lifts
In scissor lifts and similar applications, pressure sensors assist in regulating the hydraulic pressure of the primary cylinder, ensuring that the lift operates smoothly and steadily.
When used with an angle sensor, a pressure sensor can help manage the platform overload condition, satisfying ANS192 and EN280 safety standards.
In such applications, it is particularly critical to consider pressure sensor redundancy as sensor failure could potentially cause injury to human operators.
Reliability in Injection Molding
Although injection molding and scissor lifts share the same type of hydraulic system in general terms, certain parameters differ between the two applications, such as the types of fluids used, as well as the sizes of the motor and cylinder.
In injection molding, the hydraulic system is responsible for controlling the actuation of the molds as they clamp together and pull apart. The pressure sensor frequently provides feedback on the hydraulic pressure of the mold actuation, enabling the pumps to efficiently and repeatably maintain the motion.
If a sensor were to fail, it could cause mold downtime and decreased product output. However, utilizing a strong and appropriately rated pressure sensor can help to reduce the incidence of low-quality injection molded parts and extend tool life.
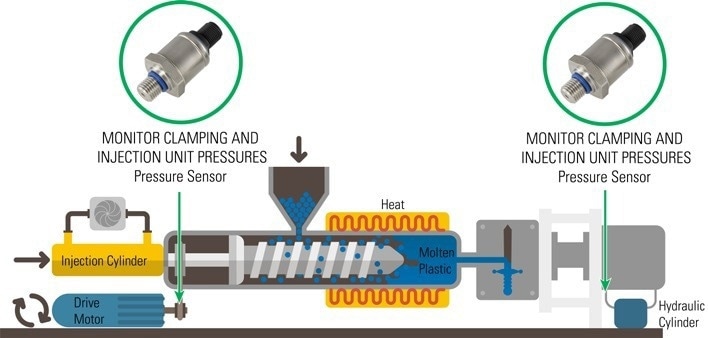
Figure 5. Using a high-quality pressure sensor in injection molding applications can help prevent downtime, minimize poor molded part quality and pro-long tool life. Image Credit: Sensata Technologies, Inc.
In hydraulic applications, where system pressures typically exceed 50 bar (725 psi), pressure sensors play a crucial role.
By ensuring safe and efficient operation, these crucial components help keep equipment running smoothly. Any failure caused by incorrect design specifications in the process can lead to dangerous working conditions or expensive downtime.
When selecting pressure sensors for hydraulic systems, design engineers must take into account the frequent challenges encountered in such applications, along with key specifications such as sensing technology, burst strength, shock and vibration, and Electromagnetic Compatibility.
Opting for components with more stringent ratings can mitigate the risk of component failure and enhance the safety and dependability of the equipment.

This information has been sourced, reviewed and adapted from materials provided by Sensata Technologies, Inc.
For more information on this source, please visit Sensata Technologies, Inc.