Superconductivity is a notable discovery that has recently gained significant attention. Conventional superconductors, such as aluminum and niobium, are considered major participants in this domain.1 However, the focus has shifted toward unconventional superconductivity.
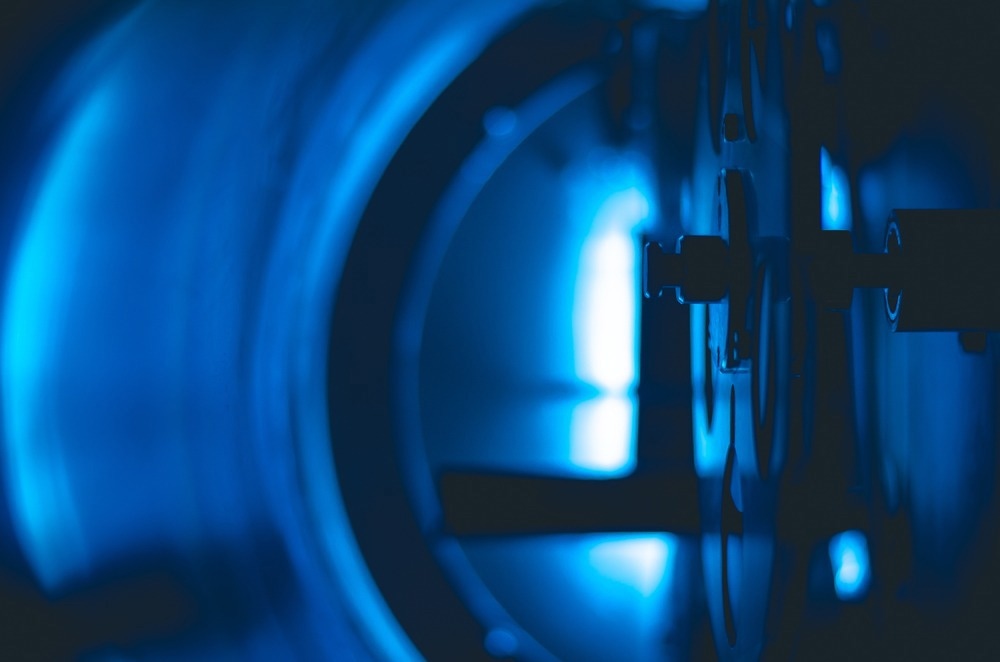
Image Credit: Dmitrii Shulgov/Shutterstock.com
Conventional Versus Unconventional Superconductivity
According to the traditional theory proposed by pioneers Bardeen, Cooper, and Schrieffer (BCS), conventional superconductivity occurs when a material's resistance drops to zero after being super-cooled to a specific critical temperature, denoted as Tc.
The magnetic field is also expelled from the superconductor due to electron-phonon coupling—an interaction between electrons and material vibrations.
Unconventional superconductivity differs from this traditional process. In this case, Cooper pairs do not conform to the standard BCS model. Instead, their attraction and the process leading to their condensation into a superconducting state may be influenced by factors such as the exchange of spin fluctuations.2
This process is useful for various applications, including high-efficiency electrical power generation and the development of lightweight electrical equipment.3 These applications leverage both naturally occurring and artificially engineered materials, underscoring their importance in modern technological advancements.
Unconventional Superconductivity in Synthetic Materials
Advancements in solid-state chemistry have accelerated the production of diverse materials with special features not typically found in nature.
A significant breakthrough was the isolation of monolayer (ML) Bi2Sr2CaCu2O8+δ (Bi2212). This achievement promises exciting possibilities for exploring the behavior of atomically thin high-temperature superconductors and creating innovative devices.
Recently, researchers employed a non-invasive optical pump-probe technique to study the unconventional superconductivity in artificially fabricated monolayer Y- Bi2212.4
During these experiments, the researchers measured the temperature dependence of the pump-probe response using a four-layer (4L) sample. They found that at a temperature of 1.6 K, the slow recovery phase lasted approximately 3.2 picoseconds (ps), which is eight times slower than the fast decay observed at 100 K.
These findings are consistent with the behavior of the superconducting (SC) and pseudogap (PG) components observed in bulk high-temperature superconductors (HTSCs), where the time constant value, denoted as τSC, was around 2.5 ps.
Using the optical method, researchers were able to investigate superconductivity in a non-invasive manner with a spatial resolution of micrometers. This approach also allowed them to determine the intrinsic Tc, which was found to be close to the bulk limit.
In another development, researchers from MIT observed new unconventional superconducting behaviors in the synthetic material iron selenide (FeSe).5
Under specific conditions, often at extremely low temperatures, certain materials change their structure, leading to the emergence of new superconducting properties.
This structural transformation is referred to as a "nematic transition," which physicists believe could provide a novel method to induce superconductivity, allowing electrons to move without resistance.
In the spin-nematic scenario, the development of nematic order is propelled by increasing orbital-selective spin fluctuations. As the temperature drops below Ts, the Fermi surface becomes more anisotropic, which amplifies the anisotropic nature of spin fluctuations.
These fluctuations, in turn, further modify the Fermi surface in a manner dependent on momentum.6 The concept of spin fluctuations stabilized by orthorhombicity is well supported by experimental data from direct investigations of spin properties.
Natural Materials Exhibiting Unconventional Superconductivity
Superconducting materials are quite uncommon. While certain elements can be found in their metallic state, superconductivity has only been observed in meteorites containing tin, lead, and indium alloys.
Recently, a team of researchers reported strong evidence of unconventional nodal superconductivity in samples of Rh17S15, also known as the mineral miassite, with a Tc of 5.4 K.
They conducted measurements of the London penetration depth using the tunnel diode resonator (TDR) technique. The pristine sample showed a clear superconducting transition at Tc = 5.31 K.
To analyze the superconducting gap structure, the researchers examined the temperature-dependent normalized superfluid density. Previous studies reported λ(0) values ranging from 490 to 790 nm. The researchers found λ(0) = 550 nm, falling between the values reported in the literature.
Despite Rh17S15 being a multiband material, its superconducting state displayed a single gap, consistent with findings from heat capacity measurements.
Unaltered Rh17S15 appears to be the first unconventional superconductor found in mineral form. However, impurities like iron, nickel, platinum, and copper, commonly found in natural minerals at low levels, make it highly improbable for them to exhibit superconductivity.
YBa2Cu3O7-x (YBCO) is a well-known unconventional superconductor, yet much remains to be discovered about its characteristics and how it responds to external factors.
One particularly interesting interaction occurs when YBCO is paired with a ferromagnet (FM).
Recent studies have explored the effects of incorporating an intermediate SrTiO3 (STO) layer in heterostructures comprising thin films of YBCO and CRO grown using pulsed laser deposition (PLD).7
The reference samples, both the pure YBCO and the YBCO/STO combination, displayed nearly identical properties, with a Tc of around 86 K. However, upon depositing CRO onto these samples, the Tc for both significantly dropped to approximately 53 K.
Interestingly, directly depositing CRO onto YBCO led to a strong ferromagnetic signal, while separating the layers resulted in reduced magnetization. The superconductivity was also notably suppressed following the direct deposition of the CRO film.
Subsequent oxidation revealed a partial recovery of the superconducting properties, likely due to effective oxygen diffusion through the STO barrier. This underscores the importance of carefully considering any modifications to unconventional superconductors and analyzing their potential impacts on the intended applications.
Importance of Machine Learning Algorithms for Unconventional Superconductors
Scientists continually develop new techniques to design efficient superconductors, a task that presents significant challenges and requires the study of numerous material properties and the analysis of vast amounts of data.
In recent years, machine learning (ML) technologies have become increasingly prominent as reliable tools for handling complex datasets.
Researchers have introduced a method for supervised classification and regression of superconducting materials that leverages Deep Sets technology.8 This family of deep learning algorithms is specifically tailored to work with sets defined by a finite enumeration of distinct members or elements.
The unique design of the modern neural network allows experts to work with sets of data rather than individual entries. This approach is crucial for accurately processing inputs that represent the chemical composition of the crystals under examination. Working with sets also helps prevent biases that could arise from specific orderings of elements in the provided list.
The implementation of modern technologies and examination methods assures a promising future for unconventional superconductors. As these methods evolve, we can expect to uncover many more secrets of superconductivity in the years to come.
More from AZoM: Magnetic Properties of Steel and Their Technological Applications
References and Further Reading
[1] Nguyen, D., et al. (2021). Superconductivity in an extreme strange metal. Nat Commun. doi.org/10.1038/s41467-021-24670-z
[2] Stewart, G. (2017). Unconventional superconductivity. Advances in Physics. doi.org/10.1080/00018732.2017.1331615
[3] Yao, C., et al. (2021). Superconducting materials: Challenges and opportunities for large-scale applications. Iscience. doi.org/10.1016%2Fj.isci.2021.102541
[4] Xiao, Y., et al. (2024). Optically Probing Unconventional Superconductivity in Atomically Thin Bi2Sr2Ca0. 92Y0. 08Cu2O8+ δ. Nano letters. doi.org/10.1021/acs.nanolett.4c00559
[5] Chu, J., (2023). Physicists discover a new switch for superconductivity. [Online] MIT News. Available at: https://news.mit.edu/2023/physicists-discover-new-switch-superconductivity-0622 (Accessed on 13 April 2024).
[6] Occhialini, C., et al. (2023). Spontaneous orbital polarization in the nematic phase of FeSe. Nat. Mater. doi.org/10.1038/s41563-023-01585-2
[7] Ionescu, A., et al. (2022). Ferromagnetism and Superconductivity in CaRuO3/YBa2Cu3O7-δ Heterostructures. Materials. doi.org/10.3390/ma15072345
[8] Pereti, C., et al. (2023). From individual elements to macroscopic materials: in search of new superconductors via machine learning. npj Comput Mater. doi.org/10.1038/s41524-023-01023-6
Disclaimer: The views expressed here are those of the author expressed in their private capacity and do not necessarily represent the views of AZoM.com Limited T/A AZoNetwork the owner and operator of this website. This disclaimer forms part of the Terms and conditions of use of this website.