By Taha KhanReviewed by Lexie CornerOct 31 2024
Solid-state sodium batteries (SSSBs) are rechargeable batteries that use solid electrolytes and sodium ions. They offer a more abundant and cost-effective alternative to lithium-based batteries. This article explores the advantages and challenges involved in SSSB development.
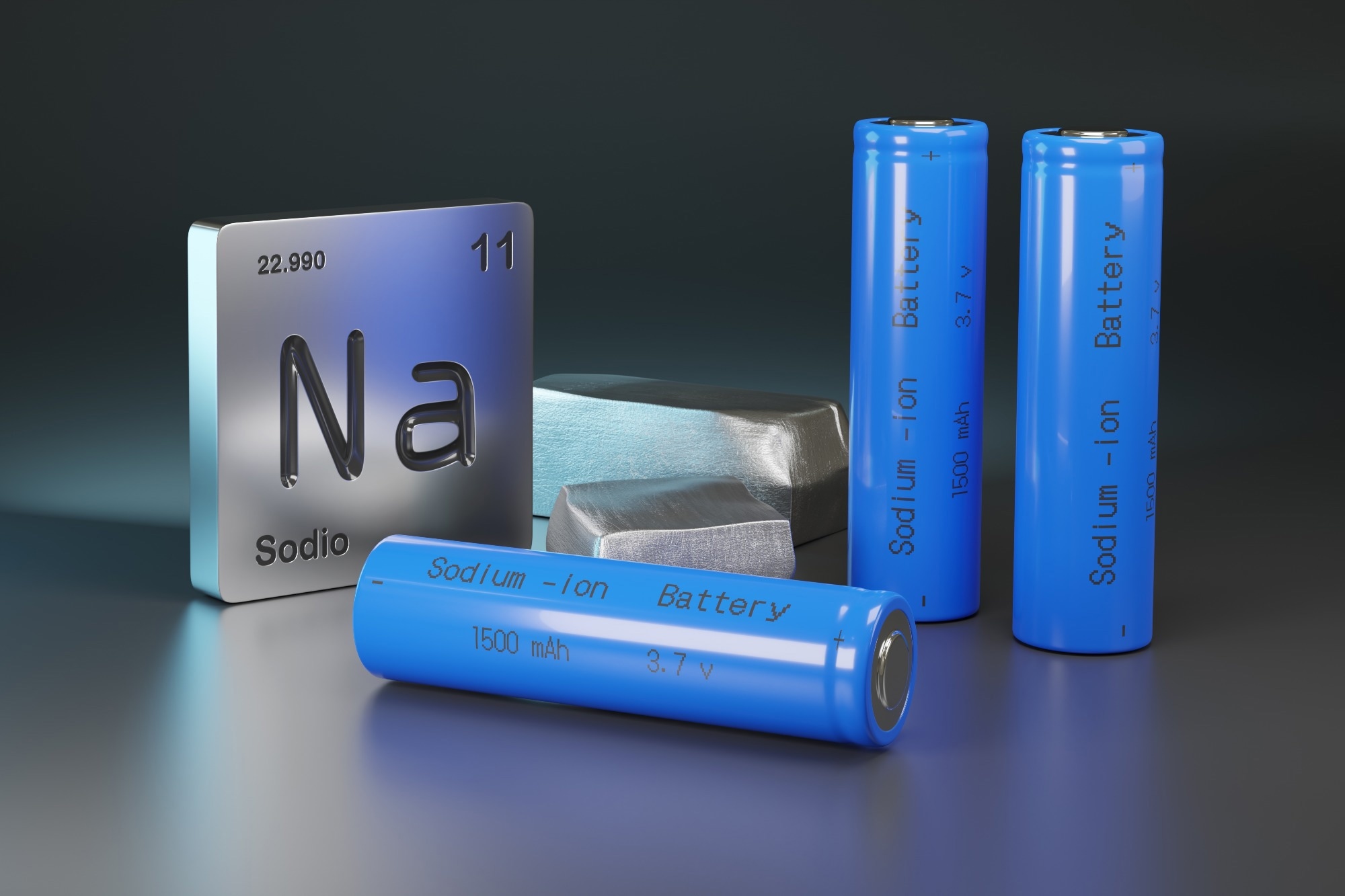
Image Credit: Juan Roballo/Shutterstock.com
Advantages
Abundant and Low-Cost Resources
Solid-state sodium batteries use sodium, a more abundant and accessible resource compared to lithium. Sodium is primarily derived from sodium chloride, which is abundant in seawater and readily available from salt deposits around the world. In contrast, lithium is relatively scarce and is extracted from limited geographic areas, often through extensive and environmentally demanding mining processes.
The abundance of sodium contributes to lower production costs, making SSSBs a cost-effective option for large-scale energy storage. This reduced cost is particularly advantageous for applications where minimizing expenses is essential, such as renewable energy storage in grid-scale operations that require numerous batteries to store and balance intermittent energy from sources like solar and wind.1, 2
Improved Safety
In liquid electrolyte batteries, flammability risks arise from the volatile organic compounds that can ignite under stress conditions, such as high temperatures, overcharging, or physical damage. SSSBs, however, use solid electrolytes that are non-flammable and considerably more stable than these organic liquids.
Solid-state electrolytes are also highly resistant to leakage and rupture, common failure points in liquid electrolyte systems. This structural stability allows SSSBs to operate safely across a wider temperature range without the elevated risk of fire or explosion.
This increased safety is valuable across many sectors, including consumer electronics, electric vehicles, and grid storage, where reducing fire and explosion risks is essential. In the automotive industry, where lithium-ion battery fires are a concern, SSSBs provide a potentially safer alternative for electric vehicle manufacturers.3, 4
High Energy Density and Long Lifespan
Solid-state sodium batteries are designed to achieve high energy density, enabling them to store more energy per unit of weight or volume compared to many conventional battery technologies.
While lithium-ion batteries are recognized for their high energy density, advancements in solid-state technology have allowed SSSBs to reach similar levels. This capability is particularly important for EV manufacturers who seek higher energy density to extend vehicle range without adding weight, making SSSBs a more sustainable solution to meet these requirements.
Additionally, the solid electrolyte offers greater resistance to wear, allowing the battery to sustain its performance and capacity over a significantly higher number of cycles.3, 4
Lithium-Ion Battery Component Characterization via SEM, EDS and ToF-SIMS in FIB-SEM Tomography
Challenges
Low Ionic Conductivity
Ionic conductivity refers to the movement of ions through the electrolyte, from the cathode to the anode during charging and in reverse during discharging. Rapid ion transfer is essential for a battery to achieve high power output and fast charging. However, most solid-state electrolytes available for sodium batteries exhibit lower ionic conductivity compared to the liquid electrolytes used in conventional batteries.5
Low ionic conductivity in solid electrolytes results from ions facing resistance as they move through the rigid, often crystalline structure of the material. This resistance slows ion transport and can limit the battery’s efficiency, making it challenging for SSSBs to compete with lithium-ion batteries, particularly in applications like electric vehicles that require high power output or rapid charge cycles.
Researchers are exploring various methods to improve ionic conductivity in solid-state sodium. In a 2021 study, scientists addressed this issue by enhancing the Na3Zr2Si2PO12 solid electrolyte. They developed an optimized Na3.4Mg0.1Zr1.9Si2.2P0.8O12 composition by substituting Zr4+ ions with Mg2+ and P5+ ions with Si4+, achieving a significant 17-fold increase in room-temperature ionic conductivity to 3.6 × 10-3 S cm-1.
This modified electrolyte also demonstrated strong resistance to dendrite formation, supporting stable sodium plating and stripping at various current densities. When applied in all-solid-state sodium batteries, the enhanced electrolyte delivered improved discharge capacities.5
Stability Limitations in Sulfide Solid Electrolytes
A key challenge in synthesizing sulfide solid electrolytes for solid-state sodium batteries is the instability of conventional sulfide starting materials, which limits compositional flexibility and complicates production. Typically, these materials require sealed reaction vessels to prevent sulfur vaporization at high temperatures, restricting operational conditions and making large-scale production more difficult.
In a 2024 study, researchers addressed this challenge by developing a synthesis method using Na₂Sₓ polysulfide flux as a stable reactant. This approach circumvents the need for unstable sulfides, allowing reactions to proceed under ambient pressure and eliminating the requirement for sealed vessels.
The Na₂Sₓ flux efficiently reacted with elemental raw materials, creating stable, stoichiometric electrolytes with high ionic conductivity in a simpler, one-step process. This method provides a versatile solution, expanding material options while maintaining high performance in solid-state sodium batteries. 6
Future Prospects
The inherent advantages of solid-state sodium batteries in terms of safety, energy density, and resource availability make them a compelling alternative to traditional lithium-ion batteries. However, significant challenges related to ion conductivity and stability limitations need to be addressed.
Future research efforts should focus on developing advanced solid electrolyte materials with high ionic conductivity and mechanical stability. Innovative manufacturing techniques are also required to ensure efficient and scalable production of solid-state batteries. As these challenges are overcome, solid-state sodium batteries have the potential to contribute significantly to a sustainable future.
To Learn More: What Are the Latest Innovations in Solid-State Battery Technologies?
References and Further Reading
- Zhao, C., et al. (2018). Solid‐state sodium batteries. Advanced Energy Materials. https://doi.org/10.1002/aenm.201703012
- Lu, Y., Li, L., Zhang, Q., Niu, Z., Chen, J. (2018). Electrolyte and interface engineering for solid-state sodium batteries. Joule. https://www.cell.com/joule/fulltext/S2542-4351(18)30339-8?sf195790525=1
- Yang, HL., Zhang, BW., Konstantinov, K., Wang, YX., Liu, HK., Dou, SX. (2021). Progress and challenges for all‐solid‐state sodium batteries. Advanced Energy and Sustainability Research. https://doi.org/10.1002/aesr.202000057
- Yao, Y., et al. (2020). Toward high energy density all solid‐state sodium batteries with excellent flexibility. Advanced Energy Materials. https://doi.org/10.1002/aenm.201903698
- Shen, L., Yang, J., Liu, G., Avdeev, M., Yao, X. (2021). High ionic conductivity and dendrite-resistant NASICON solid electrolyte for all-solid-state sodium batteries. Materials Today Energy. https://doi.org/10.1016/j.mtener.2021.100691
- Nasu, A., Otono, T., Takayanagi, T., Deguchi, M., Sakuda, A., Tatsumisago, M., Hayashi, A. (2024). Utilizing reactive polysulfides flux Na2Sx for the synthesis of sulfide solid electrolytes for all-solid-state sodium batteries. Energy Storage Materials. https://doi.org/10.1016/j.ensm.2024.103307
Disclaimer: The views expressed here are those of the author expressed in their private capacity and do not necessarily represent the views of AZoM.com Limited T/A AZoNetwork the owner and operator of this website. This disclaimer forms part of the Terms and conditions of use of this website.