Introduction
The oxide-based phosphors possess several advantages such as chemical inertness, thermal stability and anti-plasma attack characteristics. Yttrium oxide is one of the most important compounds of yttrium and accounts for the largest use. The crystalline state of Y2O3:Eu is one of the important materials for uses for both ultraviolet- and electron-exited phosphors. It is already used in manufacturing Y2O3:Eu phosphor to give the red color in color television tubes [1-8]. Although the Y2O3:Eu crystallites possess high luminescence efficiency, high quality of color purity and good chemical and thermal stability, it is important to ensure the most efficient utilization of the Eu activator in these phosphors in view of the high materials cost.
We have developed a chemical vapor deposition (CVD) technique operated under atmospheric pressure for growth of metal oxide amorphous and crystalline films. Our CVD setup is fundamentally a type of thermal CVD. However this setup possesses the abilities of design of the microarchitechture of the metal oxide crystallites, epitaxy and non-epitaxy processes with high growth rate, operation without the vacuum system [9-18]. For example, highly oriented ZnO epitaxial whiskers [9, 10] and MgO/ZnO hetero-epitaxial whiskers [17] are the product of our CVD method. These oxide whiskers have high growth rate, excellent crystallinity and uniform orientation. Highly supersaturating condition is favorable for growth of various types of metal oxide whiskers.
We had already compared photoluminescence (PL) intensities obtained from randomly oriented Y2O3:Eu polycrystalline films and <100>Y2O3:Eu fluorescent whiskers [18]. Although the PL intensity of Y2O3:Eu polycrystalline films indicated the maximum intensity at the Eu concentration of 3 at.%, the intensity of highly oriented Y2O3:Eu fluorescent whiskers kept increasing to 10 at.%. This suggests that the emission efficiency might be influenced by the orientation direction of crystals.
In this study, the Y2O3:Eu fluorescent whiskers were grown on yttrium stabilized zirconia (YSZ) using the CVD technique operated under the atmospheric pressure. The PL characteristics of the Y2O3:Eu fluorescent whiskers grown to two crystalline directions.
Experimental
The single crystal of (100) and (111) YSZ substrates were cut into 10 x 10 x 0.5 mm and then ultrasonically cleaned with deionized water for 30 min. After the treatment, the single crystal was washed with methanol and acetone. The Y2O3:Eu whiskers were prepared using an atmospheric CVD apparatus as shown in Figure 1, that was previously employed for the fabrication of the epitaxial anatase films [13-16], using titanium tetra-isopropoxide as the source complex. The reactants, yttrium dipivaloyl methanato (Y(DPM)3, yttrium tris(2,2,6,6-tetramethyl)-3,5-heptanedionate, C33H57O6Y, Showa Denko Co., quoted purity of 99.9%) and europium dipivaloyl methanato (Eu(DPM)3, europium.
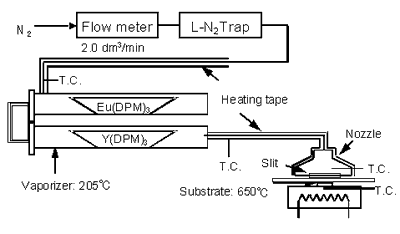
|
Figure 1. Schematic diagram of atmospheric CVD apparatus used in this study.
|
tris(2,2,6,6-tetramethyl)-3,5-heptane-dionate, C33H57O6Eu, Showa Denko Co., quoted purity of 99.7%) were loaded into a vaporizer and vaporized using an electric heater. The inside temperature of the vaporizer measured using a K-type thermocouple is defined as the vaporizing temperature. The vaporizing temperature was kept constant at 205ºC. The reactant vapor was first carried by nitrogen gas flowing at a rate of 2.0 dm3/min and then sprayed from the metallic nozzle directly onto the single crystalline (100) and (111) YSZ substrates mounted on the electric heater. The surface temperature measured using the K-type thermocouple is defined as the substrate temperature. The reactant vapor was immediately decomposed by thermal energy from the substrate heater to form polycrystalline whisker. First, the deposition duration of Y2O3:Eu whiskers synthesized on (100) and (111) YSZ substrates was varied to find out the growth rate. Next, the Y2O3:Eu crystallites were synthesized for appropriate time between 50 and 25 min for each experiment to maintain a film thickness of 10 μm. The substrate was heated to 650°C using the electric heater. The distance between the nozzle and the substrate was maintained at 15 mm throughout the experiments. The photoluminescence spectrum of the sample was measured at room temperature (using a spectrometer, FP 6500DS, JASCO Co.), equipped with a grating with 1800 grooves/mm. The wavelength resolution was held at 1 nm. The metal composition of the samples was determined using inductive coupled plasma-atomic emission spectroscopy (ICP-AES) with a spectrometer, SPS-400, Seiko Instruments Co.
Results and Discussion
To obtain the growth with preferential orientation, the Y2O3:Eu films were synthesized at the temperature region in which inhomogeneous gas reaction occurs. The preferential orientation direction of Y2O3:Eu whiskers grown on the (100) and (111) YSZ substrates has already determined to be <100> and <111>, respectively, using x-ray diffraction [19].
Figures 2 and 3 illustrate the PL spectra of the <100> and <111> Y2O3:Eu whiskers, respectively. The peaks at 593 nm, 599 nm as well as the most intense peak at 611 nm assigned to 5D0→7F2 transition [1-8] were seen on the spectra. The obtained peaks in the neighborhood of 590 nm and of 630 nm corresponding to 5D0→7F1 transition and 5D0→7F3 transition, respectively. No difference between the PL spectra obtained from the <100> and <111> Y2O3:Eu whiskers were seen. In addition, it was shown that luminescence intensities increased with increasing the Eu concentration. These results suggest that the intensity is strongly dependent upon the concentration of Eu. Figure 4 illustrates relative intensities of the prominent line at 611 nm observed from the <100> and <111> Y2O3:Eu whiskers. The value of the highest luminescence intensity is normalized to 100%. Both curves in Figure 4 exhibit linear increase with increasing the dopant concentration. The PL intensity of the <111> Y2O3:Eu whiskers is higher than that of the <100> Y2O3:Eu whiskers.
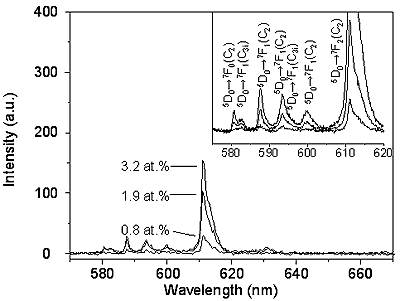
|
Figure 2: PL spectra of <100> Y2O3:Eu whiskers at various Eu concentrations.
|
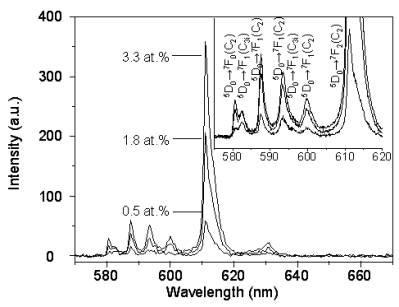
|
Figure 3: PL spectra of <111> Y2O3:Eu whiskers at various Eu concentrations.
|
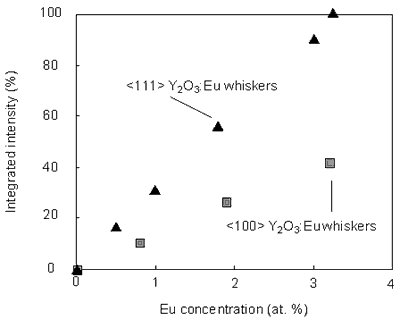
|
Figure 4: Relative integrated intensity of the peak at 611 nm.
|
It is well-known that rare-earths doped in yttria incorporate into two non-equivalent crystal sites having different symmetries of C2 and C3i. Although these sites are constructed from 6 oxygen ions and two oxygen vacancies, as shown in Figure 5, the position of the oxygen vacancies are differ between two sites. When Eu3+ ion occupies the C3i site, the emission of 7F0→5D0 transition is forbidden. On the other hand, when Eu3+ ion occupies the C2 site, the emission of 5D0→7FJ transition is allowed.
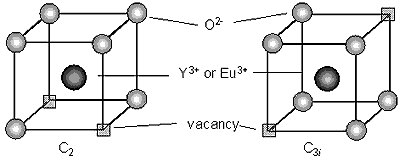
|
Figure 5: Drawing of yttrium ion site in the cubic form of Y2O3.
|
R. B. Hunt et al. [20] reproduced the energy-level scheme for the 7F0, 7F1, 5D0 and 5D1 levels of Eu3+ in Y2O3, for both C2 and C3i sites, based on the result reported by Heber et al. [21] on the single crystal of Y2O3:Eu. Following the reported data, the 5D0 level for the C3i sites is predicted to be 87 cm-1 higher in energy than the 5D0 level of the C2 sublattice. Therefore, the Eu3+ emission exhibits the luminescence peak at 587.7 nm and 582.4 nm from the C2 and C3i sites, respectively.
Taking into account this fact, we can compare an actual situation with the intensities of the above mentioned lines, in the case of these two kinds of whiskers grown on the substrates with different orientations. Relative integrated intensity ratio of the lines 587.7 nm and 582.4 nm is expected to extend unity, if there is a preferential incorporation of the Eu3+ ion in one of these sites. The result which compares the relative integrated intensity ratio of 587.7 nm and 582.4 nm lines is shown in Figure 6. With the doping, the intensity ratio of the peak at 587.7 nm to that at 582.4 nm was slightly increased for the <111>Y2O3:Eu whiskers and decreased for the <100>Y2O3:Eu whiskers, suggesting the preferential incorporation of Eu3+ in the site with symmetry C2. This is thought to be one of the possible reasons for occurrence of the luminescence intensity deference between <111> and <100> Y2O3:Eu whiskers.
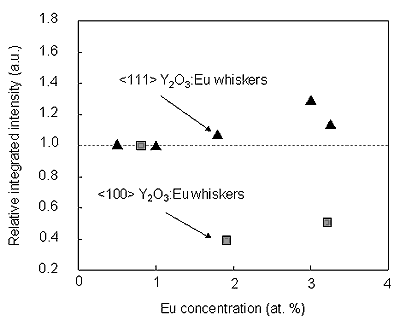
|
Figure 6: Relative integrated intensity of the peak originated from 5D0→7F1 (C2/C3i). Excitation was performed using 254 nm photons.
|
Conclusion
The <100> and <111> Y2O3:Eu fluorescent whiskers were grown on (100) and (111) YSZ using the CVD technique operated under the atmospheric pressure, respectively. The red emission obtained from the <111> Y2O3:Eu whiskers is higher than that of the <100> Y2O3:Eu whiskers. The spectrum analysis suggests the preferential incorporation of Eu3+ in the site with symmetry C2 in the <111> Y2O3:Eu whiskers. This phenomenon may enhance the red emission of the <111> Y2O3:Eu whiskers.
Acknowledgement
This work was partially supported by a Grant-in Aid for Scientific Research (B) and Exploratory Research, the Ministry of Education, Science, Sports and Culture contract Nos. 11450247 and 14655269. The authors also thank to the support by the 21st century COE program at Nagaoka University of Technology.
References
1. K.G. Cho, D. Kumar, P.H. Holloway and Rajiv K. Singh, “Luminescence Behavior of Pulsed Laser Deposited Eu:Y2O3 Thin Film Phosphors on Sapphire Substrates”, Appl. Phys. Lett., 73 (1998) 3058-3060.
2. P.K. Sharma, R.Nass and H. Schmidt, “Effect of Solvent Host Precursor, Dopant Concentration and Crystallite Size on Fluorescence Properties of Eu(III) Doped Yttria”, Optical Materials, 10 (1998) 161-169.
3. L. Ozawa, “Cathodoluminescence -Theory and Applications”, VCH publishers, New York (1990) pp. 78.
4. G. A. West and K. W. Beeson, “Low-pressure Metalorganic Chemical Vapor Deposition of Photoluminescent Eu-doped Y2O3 Films”, J. Mater. Res., 5 (1990) 1573-1580.
5. G. A. Hirata, J. Mckittrick, M. Avalos-Borja, J.M. Siqueiros and D. Devlin, “Physical Properties of Y2O3:Eu Luminescent Films Grown by MOCVD and Laser Ablation”, Appl. Surf. Sci., 113 (1997) 509-514.
6. O. P. Y Moll, A. Huignard, E. Antic-Fidancev, P. Ashehoug, B. Viana, E. Millon, J. Perrere, C. Garapon and J. Mugnier, “Eu3+- and Tm3+- Doped Yttrium Oxide Thin Films for Optical Applications”, J. Lumin., 87-89 (2000) 1115-1117.
7. S. L. Jones, D. Kumar, K.-G. Cho, R. Singh and P.H. Holloway, “Pulsed Laser Deposition of Y2O3:Eu Thin Film Phosphor”, Displays, 19 (1999) 151-167.
8. S. J. Rhee, J. O. White, S. Lee and H. Chen, “Growth and Characterization of Y2O3:Eu on Si and Yttriuma-Stabilized Zirconia”, J. Appl. Phys., 90 (2001) 6110-6113.
9. M. Satoh, N. Tanaka, Y. Ueda, S. Ohshio and S. Saitoh, “Epitaxial Growth of Zinc Oxide Whiskers by Chemical-Vapor Deposition under Atmospheric pressure”, Jpn. J. Appl. Phys., 38 (1999) L586-L589.
10. H. Saitoh, M. Satoh, N. Tanaka, Y. Ueda and S. Ohshio, “Homogeneous Growth of Zinc Oxide Whiskers” Jpn. J. Appl. Phys., 38 (1999) 6873.
11. H. Saitoh, Y. Namioka, H. Sugata, S. Ohshio, “Nanoindentation Analysis of Al:ZnO Epitaxial Whiskers”, Jpn. J. Appl. Phys., 40 (2001) 6024-6028.
12. Y. Ohkawara, T. Naijo, T. Washio, S. Ohshio, H. Ito and H. Saitoh, “Field Emission Property of Al:ZnO Whiskers Modified by Amorphous Carbon and Related Films”, Jpn. J. Appl. Phys., 40 (2001) 7013-7017
13. S. Tokita, N. Tanaka and H. Saitoh, “High-Rate Epitaxy of Anatase Films by Atmospheric Chemical Vapor Deposition”, Jpn. J. Appl. Phys., 39 (2000) L169-L171.
14. N. Tanaka, S. Ohshio and H. Saitoh, “Preferential Orientation of Titanium Dioxide Polycrystalline Films Using Atmospheric CVD Technique”, J. Ceram. Soc. Jpn., 105 (1997) 551-554.
15. H. Saitoh, H. Sunayama, N. Tanaka and S. Ohshio, “Initial Growth process of Epitaxially Chemical-vapor-deposited Titanium Dioxide Crystallites”, Mater. Sci. & Eng. Serv. Soc., (1998) 165-168.
16. H. Saitoh, H. Sunayama, N. Tanaka and S. Ohshio, “Epitaxial Growth Mechanism of Chemical-Vapor-Deposited Anatase on Strontium Titanate Substrate” J. Ceram. Soc. Jpn., 106 (1998) 1051-1055.
17. H. Saitoh, Y. Okada and S. Ohshio, “Synthesis of MgO/ZnO Hetero-Epitaxial Whiskers Using Chemical Vapor Deposition Operated under Atmospheric Pressure”, J. Mater. Sci., 37 (2002) 4597-4602.
18. Y. Satoh, S. Ohshio and H. Saitoh, “Photoluminescence Properties of Y2O3:Eu Whiskers with Preferential <100> Orientation”, Jpn. J. Appl. Phys., 41 (2002) L1253-1255.
19. Y. Satoh, H. Najafov, S. Ohshio and H. Saitoh, submission to Jpn. J. Appl. Phys.
20. R. B. Hunt Jr. and R.G. Pappalado, “Fast Excited-State Relaxation Eu-Eu Pairs in Commercial Y2O3:Eu3+ Phosphors”, J. Lumin., 34 (1985) 133-146.
21. J. Heber, K. H. Hellwege, U. Kobler and H. Murmann, “Energy levels and interaction between Eu3+-ions at lattice sites of symmetry C2 and symmetry C3i in Y2O3”, Z. Phys., 237 (1970) 189-204.
Contact Details
Yuko Satoh, Hikmet Najafov, Shigeo Ohshio and Hidetoshi Saitoh
Department of Chemistry
Nagaoka University of Technology
Nagaoka
Niigata 940-2188
Japan
E-mail: [email protected]
|