Introduction The hydrogen is expected to play an important role as a secondary energy resource because it is clean and inexhaustible. The use of the hydrogen gas involves a great risk because it has a wide range of explosive concentrations and low ignition energy, as well as its colorless and odorless nature. For that reason, a sensing device for monitoring hydrogen is required to use hydrogen safely. Conventional electrical sensors, such as semiconducting ceramics (SnO2 or ZnO) [1, 2] and simple platinum wire, must be heated up to 150-400˚C to enhance the sensitivity. Therefore, this type of sensor has a risk of ignition on account of its high operating temperature and the possibility that an electrical spark could be generated on the sensing circuit. To avoid these problems, we have developed a new type of optically readable hydrogen sensors. The principle of the optically readable sensors is based on the change in the transmittance and the reflectance of thin films such as Pd [3], Pd/WO3 [4] and Pd/MoO3 [5] on a glass substrate, due to hydride formation. These sensors have a great advantage of high safety and prevention of an explosion. A major change in the optical properties of rare-earth metals with forming their hydrides has been reported by Huiberts et al. [6]. Yttrium (Y) was selected out of the rare-earth metals as a layer detecting hydrogen. Moreover, Palladium (Pd) with outstanding hydrogen sorption was used as a layer of catalyst on the Y layer. There is the possibility that an optical sensor with high sensitivity can be realized using these materials. However, the characteristics of their materials have not been investigated from the point of view of hydrogen sensing characteristics such as response speed, repeatability and hydrogen concentration dependence of sensitivity. In this paper, the optical properties of the Pd/Y double-layered thin films were investigated. Experimental The sensing devices consisting of a Y film covered with a Pd film were prepared on a glass substrate (Corning #7059) using a R. F. magnetron sputtering apparatus (Anelva, SPF-332H), which was equipped with 99.99% Pd and 99.9% Y plates. The sputtering was carried out under 1 Pa Ar atmosphere. After the deposition of the Y film on the substrate, the Pd film was continuously formed on the Y film. The Pd film plays an important role not only in protecting the Y film against its oxidation but also in catalyzing hydrogen dissociation. To compare the Pd/Y sensor with previously [3] reported Pd-based sensor, the Pd monolayered film was also prepared through the similar procedure. A 50 nm Y film covered with a 20 nm Pd film and the Pd monolayered film were prepared so as to have the total thickness of 70 nm. Moreover, the thickness of the Y film covered with the 20 nm Pd film was varied from 20 nm to 150 nm. The transmittance and the reflectance of the Pd/Y films were measured using a double-monochromator spectrophotometer (Shimadzu, UV-365). X-ray diffraction (XRD) analysis was conducted to identify the products and its crystalline orientation using an X-ray diffractometer (RIGAKU, RINT-1500). To obtain the optical characteristics and XRD profiles of the Pd/Y films in hydrogen or air atmosphere, cells into which hydrogen or air could be introduced were attached to these equipments. For measurements of hydrogen sensing characteristics, the specimen was placed in a cell with glass windows into which air or Ar containing hydrogen could be introduced. The specimen was irradiated perpendicularly with light beam from a semiconductor laser (680 nm wavelength). The transmitted light passing through the sample was detected with a plasma monitor (Hamamatsu, PMA-10). All measurements were conducted at room temperature at 105 Pa. Results and Discussion Figure 1 shows the optical transmission spectra over the spectral range of 300-2500 nm for a 50 nm Y film covered with a 20 nm Pd film at room temperature. The transmittance of the as-deposited film with a shiny metallic state (Figure 1 (a)), which is around 1% in the visible region, remarkably increased in hydrogen atmosphere at 105 Pa (Figure 1 (b)). The change in the transmittance of the Pd/Y film was considerably greater than that of the Pd monolayered film with the identical total thickness of 70 nm. After exposure to air, the transmittance decreased (Figure 1 (c)), and the spectrum did not recover to the original one. 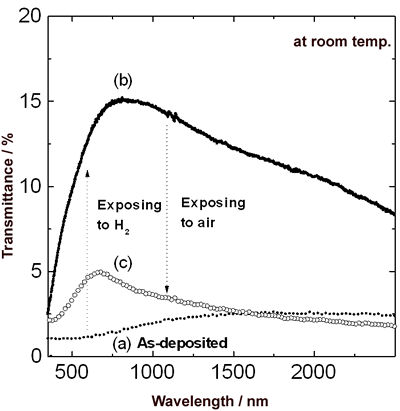 | Figure 1. Change in transmittance over the spectral range of 300-2500 nm for 50 nm Y film covered with 20 nm Pd film at room temperature. Specimens (a), (b) and (c) were as-deposited film, after exposure to hydrogen and after exposure to air respectively. | The Y film in the state shown in Figure 1 (c) lost the initial metallic gloss and turned into dark blue. Palladium efficiently catalyzes hydrogen dissociation and easily absorbs dissociated hydrogen. It is considered that hydrogen penetrated deeper into the Y film from the Pd film, and not only Pd film but also the Y film absorbed hydrogen. Figure 2 shows the reflectance over the spectral range of 250-800 nm for the Pd/Y film at room temperature. The reflectance from the Y side of the film was exhibited. The as-deposited film had highly reflective due to the free electron optical response associated with characteristics for metals. In hydrogen atmosphere, the free electrons in the Y film decreased due to a metal-insulator transition [7]. Therefore, a dramatic decrease in the reflectance was observed over 350 nm wavelength. After exposure to air, the film was highly reflective over 520 nm wavelength compared to the reflectance in hydrogen atmosphere. This is similar to the behavior of the free electrons for Y dihydrides, and the reflectance below 520 nm is governed by optical interband absorptions [8]. 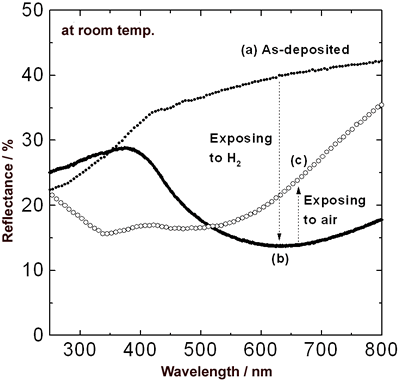 | Figure 2. Change in reflectance over the spectral range of 250-800 nm for 50 nm Y film covered with 20 nm Pd film at room temperature. Specimens (a), (b) and (c) were as-deposited film, after exposure to hydrogen and after exposure to air respectively. | To clarify the difference among each state of the transmission spectra of the Pd/Y films as shown in Figure 1 (a)-(c), we carried out X-ray diffraction analysis. For the as-deposited film (Figure 3 (a)), the peaks of Y (100), Y (002) and Pd (111) were observed. In hydrogen atmosphere (Figure 3 (b)), the peaks corresponding to Y and Pd disappeared, and the only PdH0.706 (111) peak was observed at a lower angle because the interplanar lattice spacing of Pd increased as Pd film absorbed hydrogen. The YH3-δ is formed with increasing concentration of hydrogen in the Y film [9]. In this work, however, the peaks corresponding to YH3-δ did not confirm. 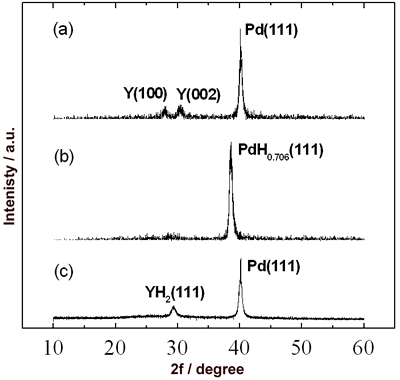 | Figure 3. X-ray diffraction profiles of 50 nm Y film covered with 20 nm Pd film. Specimens (a), (b) and (c) were as-deposited film, after exposure to hydrogen and after exposure to air respectively. | According to Huiberts et al. [6, 7], at lower hydrogen concentrations, the α-YHx (x < 0.21) is formed. At higher hydrogen concentrations, the γ-YHx (x > 2.85) is formed after the formation of the β-YHx (1.83 < x < 2.09). Increase of the hydrogen concentration leads to an abrupt and drastic increase in optical transmission intensity within the β-γ coexisting phase region. More γ-YHx phase precipitates with time. In this work, the state of the Y film in hydrogen atmosphere was considered to be the β-γ coexisting phase. However, since the thickness of the Y film (50 nm) is very thin, the peaks assigned to the β- or γ-phase did not appear in hydrogen atmosphere. After exposure to air (Figure 3 (c)), the Pd (111) was observed again, because hydrogen was excluded from the Pd hydride film. Furthermore, the peak corresponding not to Y but to YH2 (111) appeared. This result shows that hydrogen was kept in the Y film and YH2 was formed, which caused the distinction of the spectra between the states shown in Figure 1 (a) and (c). The peak of YH2 (111) disappears again and the only PdH0.706 (111) peak was observed in hydrogen atmosphere after exposure to air. Figure 4 shows the change in transmittance at a 680 nm wavelength for a 70 nm Pd film and a 50 nm Y film covered with a 20 nm Pd film as a function of time at room temperature. When the Pd film detected hydrogen, the transmittance exhibited a rapid increase to about 6 times as large as the initial value (Figure 4 (a)). After exposure to air, the transmittance returned to the initial value, and the Pd film exhibited the same sensing characteristic repeatedly. On the other hand, the transmittance of the Pd/Y film had a dramatic increase to about 17 times as large as the initial value (Figure 4 (b)), after the first detection of hydrogen. It is obvious that the Pd/Y film has a great merit of the high sensitivity compared with the Pd film. 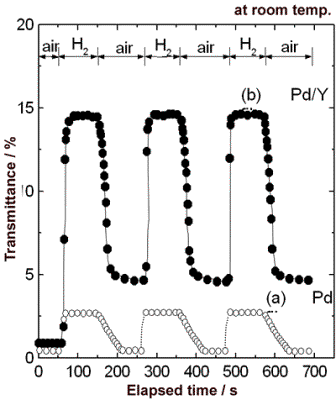 | Figure 4. Change in transmittance at a 680 nm wavelength for (a) 70 nm Pd film and (b) 50 nm Y film covered with 20 nm Pd film as a function of time at room temperature. | Furthermore, the response was very fast. While the response time of the Pd/Y film reported by Huiberts et al. was about 80 s [6], that of the film prepared in this work was 8 s or less. Moreover, the recovery time until a saturation level of transmittance was 30 s, which was shorter than that of the Pd film (about 65 s) as shown in Figure 4 (a). From the comparison described above, it is evident that the Pd/Y film has excellent response and recovery properties. After exposure to air, the transmittance did not recover to the initial value because of the YH2 formation as shown in Figure 3 (c). From the second hydrogen sensing, however, the good reproducibility was observed. This phenomenon can be utilized for a requirement of repetitious detection. Furthermore, the transmittance of the only Y film did not change at all after exposure to hydrogen gas, so that the Pd film plays an important role in catalyzing hydrogen dissociation. It is considered to be extremely important for hydrogen sensor to detect various concentrations of hydrogen. The Pd/Y film could detect 100% hydrogen as shown in Figure 4. The sensing properties under various concentrations of hydrogen were measured. Figure 5 (a) shows the change in transmittance of the Pd/Y films with detection of Ar containing 0.1-100% hydrogen at 105 Pa. The film exhibited an increase in transmittance to about 8 times as large as the initial value when the film detected 0.1% hydrogen, and the variation increased with hydrogen concentration. The variation was greater than that of the Pd film with detection of 100% hydrogen as shown in Figure 4 (a), implying that the sensitivity for low concentration of hydrogen was also high. After replacement of the entire concentrations of hydrogen by air, the transmittance decreased to the same value. Even after replacement of 0.1% hydrogen by air, the YH2 formation was confirmed by X-ray diffraction analysis. This result suggests that Y can be applied to hydrogen-storing material because the Pd/Y films can gather and keep hydrogen in the Y layer from the low concentrations of hydrogen atmosphere. It should also be noted that the film did not sense methane and isobutane at all. This fact evidences that the sensor has a high selectivity for hydrogen gas. Figure 5 (b) shows the dependence of total transmittance change (ΔT) on hydrogen pressure. The maximum transmittance change in about 13.5% was confirmed up to 5% hydrogen gas. Therefore, an upper limit for measurable hydrogen content was 5% hydrogen gas during exposure to hydrogen for 500 s. This result was similar to pressure composition isotherms of YHx in the β-γ coexisting phase region [7]. 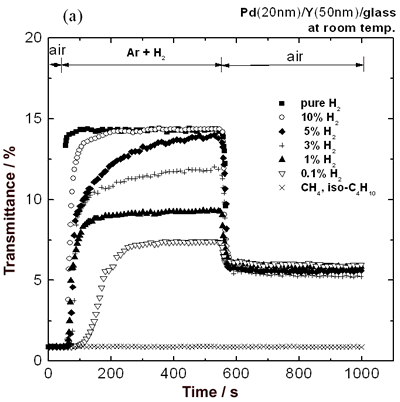 (a) 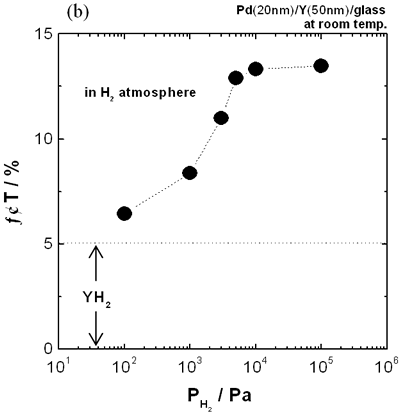 (b) | Figure 5. (a) Change in transmittance at a 680 nm wavelength for 50 nm Y film covered with 20 nm Pd film as a function of time after exposure to Ar containing 0.1-100% hydrogen, CH4 and iso-C4H10. (b) Total transmittance change as a function of hydrogen partial pressure. | Figure 6 shows the sensitivity of the Y films covered with a 20 nm Pd film and the Pd films studied by Hamagami [10] as a function of the total film thickness. The sensitivity was defined as the number obtained by dividing the transmitted intensity in hydrogen by that in air. The thickness of Y film was varied from 20 nm to 150 nm. The sensitivity of the Pd/Y films increased considerably with increasing thickness of the Y film as shown in Figure 6 (a). ![AZoJomo - The AZO Journal of Materials Online - Sensitivity of (a) Y films covered with 20 nm Pd film and (b) Pd film [10] as a function of the total film thickness. The sensitivity was defined as the number obtained by dividing the transmitted intensity in hydrogen by that in air. The thickness of Y film was varied from 20 nm to 150 nm.](/work/pAKmXBC780fNs037Q04N_files/image008.gif) | Figure 6. Sensitivity of (a) Y films covered with 20 nm Pd film and (b) Pd film [10] as a function of the total film thickness. The sensitivity was defined as the number obtained by dividing the transmitted intensity in hydrogen by that in air. The thickness of Y film was varied from 20 nm to 150 nm. | The sensitivity of the Pd films also increased slightly with the thickness as shown in Figure 6 (b). Relatively thick Pd films have a problem that the films peel off the substrate after exposure to hydrogen because of blister formation [11]. However, the thick Y films (even with 150 nm thickness) covered with a 20 nm Pd film did not entirely peel off. It was confirmed that the Pd/Y film has a great advantage not only of high sensitivity but also of high reliability. Conclusions An optically readable hydrogen sensor consisting of a Y film covered with a thin Pd film was developed. The Pd/Y film indicated a dramatic increase in transmittance to about 17 times as large as the initial value with detection of 100% hydrogen at 105 Pa. The change occurred within 8 s, which was much faster than that reported by Huiberts et al. [6]. This sensor has a quick response with detection of hydrogen at room temperature. Furthermore, the sensor exhibited a considerable increase in transmittance to about 8 times even with a detection of 0.1% hydrogen. These sensitivities of the Pd/Y film were much higher than previously reported Pd-based hydrogen sensor [3]. The change in transmittance was constant after exposure to hydrogen once. In case of repeated sensing, an excellent reproducibility of the sensor was provided. Moreover, relatively thick Y films covered with a 20 nm Pd film did not peel off the substrate after exposure to hydrogen. The Pd/Y film shows great advantage not only of high sensitivity but also of high reliability. Acknowledgements This study was partially supported by Grants for Science Research (No. 13305060) and 21st Century COE Program “Creation of Hybridized Materials with Super-Functions and Formation of International Research and Education Center” from the Ministry of Education, Culture, Sports, Science and Technology of Japan. References 1. T. Seiyama, A. Kato, K. Fujiishi and M. Nagatani, “A New Detector for Gaseous Components using Semiconductive Thin Films”, Anal. Chem., 34 (1962) 1502-1503. 2. T. Seiyama and S. Kagawa, “Study on a Detector for Gaseous Components using Semiconductive Thin Films”, Anal. Chem., 38 (1966) 1069-1073. 3. Y. Oh, J. Hamagami, Y. Watanabe, M. Takata and H. Yanagida, “A Novel Palladium Thin Film Hydrogen-Detector”, J. Ceram. Soc. Japan, 101 (1993) 618-620. 4. Y. Oh, J. Hamagami, Y. Watanabe and M. Takata, “Preparation and Characterization of an Optically-Detectable Hydrogen Gas Sensor Consisting of Pd/WO3 Thin Films”, Sensors and Actuators B, 13-14 (1993) 547-548. 5. J. Hamagami, Y. Oh, Y. Watanabe and M. Takata, “Preparation and Characterization of an Optically Detectable H2 Gas Sensor Consisting of Pd/MoO3 Thin Films”, Sensors and Actuators B, 13-14 (1993) 281-283. 6. J. N. Huiberts, R. Griessen, J. H. Rector, R. J. Wijngaardeen, J. P. Dekker, D. G. de Groot and N. J. Koeman, “Yttrium and Lanthanum Hydride Films with Switchable Optical Properties”, Nature, 380 (1996) 231-234. 7. R. Griessen , J. N. Huiberts, M. Kremers, A. T. M. van Gogh, N. J. Koeman, J. P. Dekker and P. H. L. Notten, “Yttrium and Lanthanum Hydride Films with Switchable Optical Properties”, J. Alloys Comp., 253-254 (1997) 44-50. 8. A. T. M. van Gogh and R. Griessen, “Dihydride Transmission Quenching in Switchable La1-zYzHx Mirrors”, J. Alloys Comp., 330-332 (2002) 338-341. 9. J. N. Huiberts, J. H. Rector, R. J. Wijngaarden, S. Jetten, D. de Groot, B. Dam, N. J. Koeman, R. Griessen, B. Hjorvarsson, S. Olafsson and Y. S. Cho, “Synthesis of Yttriumtrihydride Films for Ex-situ Measurements”, J. Alloys Comp., 239 (1996) 158-171. 10. J. Hamagami, “Study on Optically Readable Hydrogen Sensor”, Ph.D. Thesis, Ngaoka University of technology, Japan (1996). 11. J. Hamagami, Y. Imai, B. Huybrechts and M. Takata, Sensors and Actuators B, to be published. Contact Details Yuji Imai Department of Electronic Control Engineering Sendai National College of Technology 4-16-1 Ayashi-chuo Aoba-ku, Sendai 989-3128 Japan | Yoshiki Okuhara Japan Fine Ceramics Center (JFCC) 2-4-1 Mutsuno Atsuta-ku, Nagoya, 456-8587 Japan | Yuji Noguchi Institute of Industrial Science The University of Tokyo 4-6-1 Komaba Meguro-ku, Tokyo 153-8505 Japan | Masasuke Takata Department of Electrical Engineering Nagaoka University of Technology 1603-1 Kamitomioka-cho Nagaoka, Niigata 940-2188 Japan Email: [email protected] | |