Introduction
There is a large interest in light emitting diodes based on conjugated polymers. This comes mostly from an industrial point of view, as these materials could lead to cheap flat color displays or light sources. In particular, poly (p-phenylenevinylene) (PPV) type polymers show good electrical conductivity and photoluminescence[1-3]. They have been considered as suitable materials for light-emitting diodes (LEDs). After the first report of a polymer-containing LEDs based on poly (p-phenylenevinylene) (PPV) in 1990, a wide variety of PPV materials has been synthesized[4-5]. The rigid, conjugated backbone causes these polymers to be insoluble and nonprocessible. One approach to avoid solubility problems of rigid systems is to attach long and flexible side chains to the polymer backbone[6]. However, theπ-conjugation of the chain will be severely disturbed by the enhanced twist angle inferred by the side groups. Introducing liquid crystalline groups into the side chain of the conjugated polymers can improve not only their solubility but also the coplanarity of the main chain and afford a higher electrical conductivity and a notable electrical and optical anisotropy.This is because the polymer main chain can be aligned by the virtue of spontaneous orientation of liquid crystalline side chains and further by macroscopic orientation of liquid crystalline domains under external force, such as sheer stress and electric or magnetic field. Spontaneous orientation and externally forced orientation of the liquid crystalline side chains enable us to control electrical and optical properties as well as their anisotropies [7-11]. Taking advantage of the macroscopic order inherent to liquid crystal mesomorphism, the polymer backbone would be easily oriented in the mesophase. As a result, the liquid crystalline conjugated polymers show linearly polarized emission. These kinds of polymers, combining efficient polarization of light and the production of bright colours, would be particularly useful as backlight for conventional liquid crystalline displays because they could make the polarizer (with its high cost, weight, and low power efficiency) redundant [12-14].
In order to get soluble poly (p-phenylenevinylene) (PPV) type polymers with linearly polarized dichroism, we have synthesized shish-kebab-type liquid crystalline poly (p-phenylenevinylene)s. Different from the common side chain liquid crystal polymers, in shish-kebab-type liquid crystal polymers, the bonding of the mesogenic units to the main chain backbone is not at the end but at the center of gravity of the mesogenic units. The mesogenic unit acts as the “kebabs” and the polymer chain as the “shish” or “skewer” (the “shish-kebab”). This mean that the orientation of the mesogenic units has a stronger effect on the alignment of the polymers than the common side chain liquid crystalline polymers does. Thus, this kind of polymer should show large optical anisotropy. In this research, the relationship between the structures and their liquid crystalline properties was investigated. The optical properties of the polymers in isotropic and mesophases were elucidated.
Experiment
Materials
All reactions were carried out under an argon atmosphere. All solvents used in the reactions were distilled from appropriate drying agents under argon gas before use. The 4-(Alkyloxy) benzoic acids were recrystallized from alcohol. The other reagents were used as received.
Synthesis
The synthesis of monomers and poly {2,5-bis [(4-n-alkoxyl) benzoyloxy] 1,4-phenylenevinylene} s is summarized in Scheme 1.
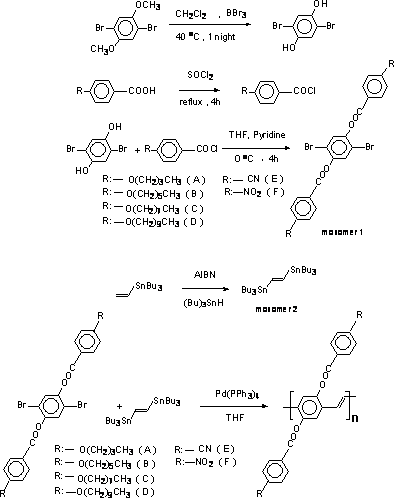
Scheme 1. Synthesis of 2,5-bis [(4-n-alkoxyl )benzoyloxy] 1,4-dibromobenzene (monomer 1).
Synthesis of 2,5-bis [(4-n-alkoxyl )benzoyloxy] 1,4-dibromobenzene (monomer 1)
The monomer 1 was synthesized according to a procedure described elsewhere [10].
Taking monomer 1-C as an example: Yield, 71% yield, melting point Tm 120°C, isotropization temperature Ti 150°C. IR (cm-1, KBr): 3089, 2951, 2853, 1725, 1600, and 1509. 1H-NMR (CDCl3) : δ= 8.10 (m, 4H , aromatic protons), 6.84 (m, 4H aromatic protons), 6.68 ( s, 2H, aromatic protons), 3.98 (t, J = 6.5 Hz, 4H,-CH2-O-), 1.77-0.82 (m, 28H, -CH2-). 13C-NMR (CDCl3) :δ=164.11, 146.97, 133.04, 115.34, 77.54, 32.19, 29.65, 26.37, 23.05 and 14.49. Elemental analysis: Calc. for C36H44Br2O6 (%): C, 71.26, H, 7.64; Found (%): C, 71.28, H, 7.62.
The monomer 1-A, 1-B, 1-D , 1-E and 1-F were prepared in a similar manner giving the following results (yield; Tm, Ti of the mesophase if any, both in °C). monomer 1-A (75% yield, 165, 170). monomer 1-B (73% yield, 145, 155). monomer 1-D (72% yield, 100, 134). monomer 1-E ( 76% yield, 226, 283). monomer 1-F (78 % yield, 245, 294).
Synthesis of 1,2-bis (tributylstannyl) ethylene
15.0 g (47.7 mmol) tributylethynyltin, 17.0 g (58.4 mmol,) tributyltinhydride, 0.21 g(0.4 mmol,)2,2'-azobis(2-isobutyronitrile) and a stir bar were charged into an Schlenk-flask under argon atmosphere. After stirring for 6 h at 90°C, the residue was distilled under reduced pressure to yield 23.6 g colorless liquid in 81.7% yield.
1H NMR (CDCl3) : δ= 5.11 (s, 2H ,double bond protons), 1.37-0.96 (m, 54H, -CH2-).13C- NMR (CDCl3) : δ= 119, 30.1, 27.2 and 11.0 Elemental analysis: Calc. for C26H56Sn2 (%): C, 51.52, H, 9.31 Found (%): C, 51.28, H, 8.88.
Polymerization
Taking polymer C as an example: 0.513 g (0.7 mmol) monomer 1-C with 5 mL DMF and 0.0238 g (0.021 mmol) tetrakis (triphenyphosphine) palladium (0) [Pd (PPh3) 4] with 45 mL DMF were charged into an Schlenk-flask under argon atmosphere. After stirring for 1 hour at 80°C, 0.42 g (0.7 mmol) 1,2-bis(tributyl tin)ethylene with 15 mL DMF was added. The mixture was heated for 96 h at 85°C, then cooled. the mixture poured into 1000 mL methanol and dilute HCl (16% percent) 20 mL, producting a precipitate.The precipitate was washed with 500 mL methanol for 24 hours, dried under vacuum, and the polymer was obtained in 91% yield.
The polymers A, B, D, E and F were prepared in a similar manner.
Characterization
FT-IR spectra were recorded on a JASCO FT/IR 8000 spectrometer using KBr discs. NMR spectra were recorded on a 500 MHz FT-NMR using CDCl3 as a solvent and TMS as an internal standard for both 1H and 13C resonance measurements. Molecular weights were determined using gel permeation chromatography (GPC) with a Shodex A-80M column using THF as eluent. DSC thermograms were obtained by a EXSTAR 6000 apparatus at a heating rate of 5°C /min under an argon atmosphere. Liquid crystalline textures were examined under crossed polarizers by using a Nikon polarizing microscope equipped with a Linkam THMS 600 hot stage. XRD measurements were performed with a Rigaku D-3F diffractometer, in which X-ray power and scanning rate were set at 1200 mW and 5° /min, respectively. UV-visible absorption spectra were measured with a Hitachi U-3500 Spectrometer. The fluorescence spectra were measured with a JASCO FP-750 spectrofluorometer. A dilute solution of the polymer (10-4 mmol in 10 mL CHCl3=10-5 M polymer solution) was droping onto quartz substrate using medicine dropper of 2 mm diameter and the solvent allowed to evaporate at room temperature for one day to give cast film. The thickness of the film was ruled by controlling the Absorbance (a.u) of the film around one unit using Hitachi U-3500 Spectrometer. the cast films were heated up to a liquid crystalline state using a polarizing optical microscope (POM) with a hot stage at a heating rate of 5°C /min, when the strong birefringence was observed, the samples was heated at this temperature for further 20 min, then cooled to a solid state quickly under atmosphere of liquid nitrogen, an annealed film was resulted.
Results and Discussion
The polymers with alkoxyl groups in the terminal sites of the side chains can dissolve in ordinary organic solvents such as THF and chloroform but the polymers with cyano and nitro groups in the end of the mesogenic units have poor solubility in ordinary organic solvents. Their molecular weights (measured using GPC) and phase transition temperatures (measured using POM and DSC) are collected in Table 1. It can be seen from Table 1 that the molecular weights of the polymers are not so high. The number-average molecular weight of the polymers with alkoxyl group is in the range of 3300 – 5500, corresponding to oligomers of 7 to 8 units. The reason for the low molecular weight is probably due to the steric hindrance occurring in the polymerization of the monomers. In spite of the low degree of polymerization, the thin cast films were easily formed from THF or chloroform solution. On the other hand, the polymers with cyano and nitro groups do not form solid films from THF or chloroform solution because of their low solubility and low molecular weight. Their low molecular weight is probably due to the low solubility of the monomers and the polymers in ordinary organic solvents.
Table 1. Thermal properties and molecular weights of the copolymers.
|
A
|
285
|
>350
|
3.3
|
6.3
|
1.9
|
6.8
|
B
|
273
|
>350
|
3.9
|
6.2
|
1.6
|
7.2
|
C
|
260
|
>350
|
4.9
|
8.3
|
1.7
|
8.2
|
D
|
250
|
>350
|
5.5
|
9.9
|
1.8
|
8.4
|
E
|
320
|
>350
|
1.9
|
2.7
|
1.4
|
4.8
|
F
|
324
|
>350
|
2.0
|
2.6
|
1.3
|
4.6
|
Tm melting point, Ti isotropization temperature (measured by POM and DSC ), D.P degree of polymerization, Mn number-average molecular weight, Mw weight-average molecular weight.
Thermotropic liquid crystalline properties of the polymers were evaluated by means of DSC measurements and texture observations using a polarizing optical microscope (POM) with a hot stage. Upon heating the polymers above their melting point, they turned into liquid crystal phases, Weak birefringence was observed, which increased with temperature. In the case of polymer D, a typical smectic fan texture (see Figure 1) was observed at 300°C. The textures of other polymers (see polymer C in Figure 2) were not so characteristic, even at temperatures up to 350°C, which is the limit temperature of our instrument. The melting point (Tm) of the polymers decreases when the length of the alkoxy tails of the mesogenic units increases.
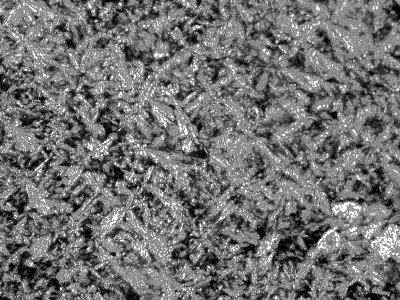
Figure 1. Broken fan texture of the polymer D taken at 300°C in heating process (magnification: ×200).
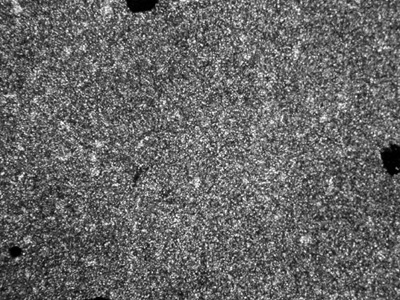
Figure 2. POM photo of the polymer C taken at 320°C in heating process (magnification: ×200).
In order to identify the mesophase of the polymers, the X-ray diffraction study of a powdery sample of the polymers was conducted. A small amount of polymers was heated to the liquid crystalline state on the hot stage of a polarizing optical microscope at a heating rate of 5°C/min, When strong birefringence was observed, the samples was heated at this temperature for further 20 min and then plunged into ice salt water, the sample was then washed with distilled water and dried under vacuum to give the quenched polymer. The XRD profiles of the polymers quenched from their liquid crystalline states are showed in Figure 3. All the polymers show an XRD pattern consisting of a low-angle peak and a high-angle peak. The results are collected in Table 2.
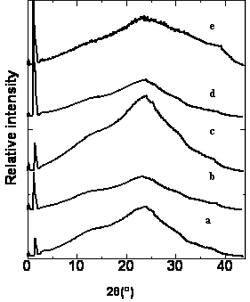
Figure 3. X-ray diffraction pattern of the polymers a: polymer A, b: polymer B, c: polymer C d: polymer D and e: polymer E.
Table 2. X-ray diffraction analysis data of the polymers.
|
A
|
1.27
23.26
|
6.96
0.38
|
2.58
|
2.70
|
SA3
|
B
|
1.55
22.80
|
5.69
0.39
|
3.04
|
1.87
|
SA2
|
C
|
1.50
23.45
|
5.87
0.38
|
3.53
|
1.66
|
SA2
|
D
|
1.41
23.26
|
6.25
0.38
|
4.00
|
1.56
|
SA2
|
E
|
1.50
23.97
|
5.86
0.37
|
1.87
|
3.13
|
SA3
|
L the most-probable length of the rodlike mesogenic units was calculated by molecular mechanical calculation using software package Spartan’02.
The low–angle Bragg reflections correspond to the thickness of the smectic layers and the high-angle peaks are related to the average distance between the mesogens within the layers [15-17]. Polymer D exhibits one low–angle Bragg reflection at 2θ= 1.41˚corresponds to a layer spacing of d = 6.25 nm,which is 1.56 times of the most-probable length of the rodlike mesogenic units (L = 4.00 nm) calculated by molecular mechanical calculation using software package Spartan’02. The results suggest that the mesophase of the polymer D is SA2 phase in which the alkoxy tails are interdigitated in an antiparallel fashion as shown in Figure 4. Similarly, polymer B and polymer C have the same SA2 phase as that of the polymer D. Polymer A and polymer E exhibit the SA3 phase.
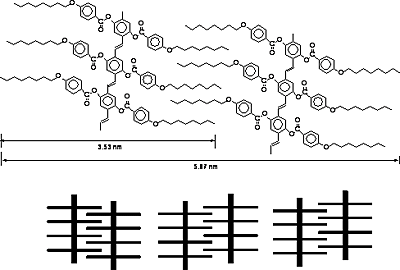
Figure 4. Speculative model showing an SA2 phase of polymer C.
UV-vis absorption spectra of polymers have two absorption bands at 266 and 364-438 nm in chloroform solution. These bands are assigned to theπ-π* transition of the mesogenic units of the side chain and that of the conjugated main chain, respectively. Figure 5 shows the UV-vis absorption spectra of polymers in chloroform.
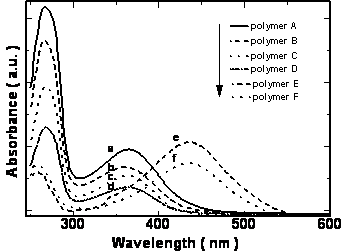
Figure 5. UV-vis spectra of polymers in chloroform a: polymer A, b: polymer B, c: polymer C, d: polymer D e: polymer E and f: polymer F.
The position of the maximum absorption peak and the general shape of the curves barely change with the increasing the length of the alkoxy tails of the mesogenic units, but the polymers with cyano and nitro groups in the terminal sites of the side chain show absorbances at longer wavelengths than those polymers with the electron donor groups. Namely, introducing the electron-withdrawing group into the mesogenic units may decrease the energy gap between the valence and the conduction band of the present conjugated polymers. The maximum absorption peak of the polymers also has large red-shift when the polymers are in different states. For example, polymer D had an absorption peak around 364 nm in chloroform solution whereas in the cast film, polymer D had a new shoulder absorption band around 450 nm due to the π-π stacking structures arising in the cast film. The planar shape of the conjugated polymer allows it to easily arrange into a co-facial π-stacking “sandwich structure”, resulting in a more coplanar conformation, hence enhancing the effective conjugation length. As a result, the position of the corresponding absorption peak is red shifted [18-19].When the cast films were heated to a liquid crystalline state and then cooled to a solid state quickly, an annealed film resulted. The annealed film prepared this way had an absorption peak at 387 nm and a new shoulder absorption band around 450 nm. It is evident that the absorption peak of the annealed polymer shifts to long wavelength, from 364 nm for cast film to 387 nm for the annealed film, indicating a longer effective conjugation length compared with the cast film. This result is expected because it is known that the liquid crystalline side chains tend to have long-range orientational order and partial positional order when the polymer in a mesomorphic state[20]. The spontaneous orientation of liquid crystalline side chains enhances the coplanarity of the main chain. The same red-shifts are also observed in the fluorescence spectra. Figure 6 and Figure 7 show the UV-vis absorption spectra and fluorescence spectra of the polymer D in different states. The polymer D has strong blue fluorescence in chloroform solution. Its cast film and annealed film have maxima shifted to longer wavelength and show yellow fluorescence. The backbone of the polymer chain is most likely a planar conformation due to the interchain interactions both in the cast film and the annealed films, the more planar the polymer chain is, the easier it is for electrons to delocalize over the polymer chain and lower the band gap between the valence and the conduction bands, which consequently causes the red shift in emission spectra[21-22]. This fact indicate that the shish-kebab structure has a strong effect on enhancing the coplanarity of the polymer main chain. Other polymers show the same phenomena as those of the polymer D. The optical properties of their isotropic and mesophases are summarized in Table 3.
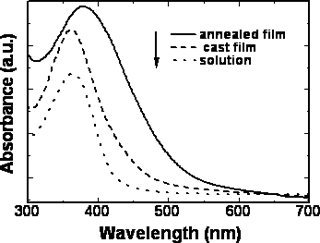
Figure 6. UV-vis spectra of polymer D in different states.
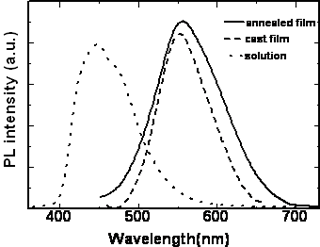
Figure 7. Fluorescence spectra of polymer D in different states.
Table 3. Optical properties of the polymers.
|
A
|
268, 364
|
363
|
382
|
445 (360)
|
552 (420)
|
554 (420)
|
B
|
268, 364
|
363
|
387
|
445 (360)
|
553 (420)
|
554 (420)
|
C
|
268, 365
|
364
|
388
|
446 (360)
|
553 (420)
|
555 (420)
|
D
|
269, 364
|
363
|
387
|
446 (360)
|
552 (420)
|
555 (420)
|
E
|
263, 438
|
|
|
505 (440)
|
|
|
F
|
262, 438
|
|
|
505 (440)
|
|
|
Conclusion
In order to develop luminescent polymer materials with linearly polarized dichroism, we have synthesized shish-kebab type liquid crystalline poly (p-phenylenevinylene) derivatives using the Stille coupling reaction. The polymers with alkoxy groups were soluble in common organic solvents. They showed liquid crystal behavior and were confirmed to form the smectic phase by X-ray diffraction studies. The melting point (Tm) of the polymers decreases when the length of the alkoxy tails of the mesogenic units increases. The polymers showed strong blue fluorescence in chloroform solution. Both their cast films and annealed films showed yellow fluorescence. The fact that the annealed film shows a larger red shift than that of the chloroform solution, both in UV-vis absorption spectra and fluorescence spectra, implies that the shish-kebab structure has strong effect on enhancing the coplanarity of the polymer main chain.
Acknowledgements
This work was supported by China Scholarship Council and Grant-in-Aids for Scientific Research from Ministry of Education, Culture, Sports, Science and Technology, Japan.
References
1. T. A. Skotheim, “Handbook of Conducting Polymers”, Marcel Dekker, New York, 1986, Vols.I-II.
2. T. A. Skotheim, R. Elsenbaumer and J. R. Reynolds, “Handbook of Conducting Polymers”, Marcel Dekker, New York, 1997, 2nd ed.
3. H. S. Nalwa, “Handbook of Organic Conducting Molecules and Polymers”, John Wiley, Chichester, 1997, Vol. 2-4.
4. M. Hay and F. L. Klavetter, “Aliphatic Phenylene Vinylene Copolymers: Tuning the Color of Luminescence through Co-monomer Feed Ratios”, J. Am. Chem. Soc., 117 (1995) 7112-7118.
5. P. F.van Hutten, J. Wildeman and A. Meetsma, “Molecular Packing in Unsubstituted Semiconducting Phenylenevinylene Oligomer and Polymer”, J. Am. Chem. Soc, 121 (1999) 5910-5918.
6. M. J. Moroni, L. Moigne and S. Luzzati, “Rigid Rod Conjugated Polymers for Nonlinear Optics: 1. Characterization and Linear Optical Properties of Poly(aryleneethynylene) Derivatives”, Macromolecules, 27 (1994) 562-571.
7. K. Akagi. and H. Shirakawa, “The Polymeric Materials Encyclopedia. Synthesis, Properties and Applications”, CRC Press, 1996, Vol.5, pp. 3669-3882.
8. K. Akagi and H. Shirakawa, “Current Trends in Polymer Science”, Alexander, J. C. 1997, Chap. 2, pp.107-112.
9. K. Akagi, and H. Shirakawa, in D.L.Wise, et al (eds.), “Electrical and Optical Polymer Systems: Fundamentals, Methods,and Applications”, Marcel Dekker, 1998, 28, pp. 983-1006.
10. S. J. Zheng, K. Akagi, Q. Xu, Z. F. Li, S. K. Cao and Q. F. Zhou, “Synthesis and Properities of Shish-kebab-type Liquid Crystalline Poly(p-phenylene)s”, Chinese J. Polym. Sci., 3 (2006) 265-271.
11. K. Akagi, H. Goto and H. Shirakawa, “Synthesis and Properties of Liquid Crystalline Conjugated Polymers”, Kobunshi Ronbunshu, 56 (1999) 217-233.
12. C. Weder, C. Sarwa, A. Montali, C. Bastiaansen and P. Smith, “Incorporation of Photoluminescent Polarizers into Liquid Crystal Displays”, Science, 279 (1998) 835-837.
13. A. Montali, C. Bastiaansen, P. Smith and C. Weder, “Polarizing Energy Transfer in Photoluminescent Materials for Display Applications”, Science, 392 (1998) 261-264.
14. M. Grell and D. D. C. Bradley, “Polarized Luminescence from Oriented Molecular Materials”, Adv. Mater., 11 (1999), 895-905.
15. P. Mariani, F. Rustichelli and G. Torquati, “Physics of Liquid Crystalline Materials”, Gordon & Breach Science, New York, 1991, Chapter 1.
16. L. V. Azaroff, “X-Ray Diffraction by Liquid Crystals”, Mol. Cryst. Liq. Cryst., 60 (1980) 73-98.
17. A. D. Vries, “The Use of X-Ray Diffraction in the Study of Thermotropic Liquid Crystals with Rod-Like Molecules”, Mol. Cryst. Liq. Cryst., 131 (1985) 125-145.
18. T. Yamamoto, D. Komarudin, M. Arai, B.-L. Lee, H. Suganuma, N. Asakawa, Y. Inoue, K. Kubota, S. Sasaki, T. Fukuda and H. Matsuda, “Extensive Studies on -Stacking of Poly(3-alkylthiophene-2,5-diyl)s and Poly(4-alkylthiazole-2,5-diyl)s by Optical Spectroscopy, NMR Analysis, Light Scattering Analysis, and X-ray Crystallography”, J. Am. Chem. Soc., 120 (1998) 2047-2058.
19. J. Cornil, D. A. dos Santos, X. Crispin, R. Silbey and J. L. Brédas, “Influence of Interchain Interactions on the Absorption and Luminescence of Conjugated Oligomers and Polymers: A Quantum-Chemical Characterization”, J. Am. Chem. Soc., 120 (1998) 1289-1299.
20. D. Demus, J. W. Goodby, G. W. Gray, H. W. Spiess and V. Vill, “Handbook of Liquid Crystals”, Wiley-Vch Verlag GmbH, Weinheim, 1998, Vols I-III.
21. A. P. Monkman, M.. Halim, I. D. W. Samuel and L. E. Horsburgh, “Protonation Effects on the Photophysical Properties of Poly (2,5-pyridine diyl)”, J. Chem. Phys, 109 (1998) 10372-10378.
22. S. F. Wang, P. P. Wu and Z. W. Han, “Photophysical Properties of Lyotropic Liquid Crystalline Polybenzazoles and Evidence of Aggregate Formation for Polybenzazoles in Methanesulfonic Acid”, Macromolecules, 36 (2003) 4567-4576.
Contact Details
Shi-Jun Zheng, Hong-Wei Shi, Xiao-Hui Chen and Shao-Kui Cao
College of the Material Science and Engineering
Zhengzhou University
Zhengzhou 450052
China
E-mail: [email protected]
|
Kazuo Akagi
Tsukuba Research Center for Interdisciplinary Materials Science (TIMS)
Institute of Materials Science, Center for Tsukuba Advanced Research Alliance (TARA)
University of Tsukuba
Tsukuba 305-8573
Japan
|
Qi-Feng Zhou
Department of Polymer Science and Engineering
College of Chemistry and Molecular Engineering
Peking University
Beijing 100871
China
|
|
|