Scientists have combined hydrogen (H2) and deuterium (D2) to produce a new “Phase IV” material in which molecular interaction was found to be different than previously observed. This is the first time Anderson localization from vibrational energy has been noticed during interaction with different masses in a material.
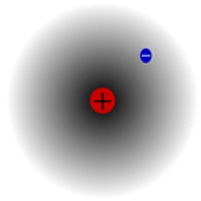
Hydrogen reacts differently to high temperature and pressure and is found abundantly in the universe. At room temperature, this element is a two-atom molecule in the gaseous state. In the Phase IV material, the hydrogen molecules displayed two different behaviors: one interacted strongly with the adjoining molecules, while the other bonded weakly with the neighboring molecules. This resulted in unique hexagonal atomic sheets similar to graphene. In electronic context, these layers behave like semimetals and semiconductors.
When producing the new hydrogen mixture, the scientists combined experiments and theoretical calculations to prevent segregation of the D2 and H2 molecules between weakly and strongly bounded layers. There was also the possibility that the molecules may be distributed in a random manner and this random state may influence the waves of atomic vibrations and prevent the molecules from propagating freely.
Based on this hypothesis, the scientists mixed H2 and D2 in different concentrations and subjected them to different level of pressures, from 2,000 times atmospheric pressure to 2.7 million atmospheres.
Raman spectroscopy was also used to validate the experiment. It was observed that the vibrational waves tend to exhibit Anderson localization when there is more than 1.9 million atmospheres.
Anderson localization is a phenomenon wherein the disordered state affects atomic vibration waves and prevents their free propagation. Also, the degree of this localization not only depends on the concentration of D2 and H2, but also on the strong or weak bounded layers of the H2 and D2 molecules.
For example, in one layer, H2 molecules at frequencies very weakly dependent on the surrounding environment vibrate in separate two to three molecule groups. The increase in hydrogen concentration caused the H2 clusters to grow and they started coupling. The latest breakthrough could prove useful in controlling thermoelectric and superconducting characteristics of new hydrogen-bearing materials.
The research has been published in Physical Review Letters.
References