Scientists at the University of Pennsylvania have now revealed a common characteristic extending through the range of glassy materials. They show that the scaling between the stiffness and strength of a glassy material does not change, implying a steady critical strain, which these materials can withstand before catastrophic failure, in spite of the high variation found in the physical properties of this class of materials.
The researchers' experiments involved measuring the strain on micropillar as it was pressed on by a punch.
Glass is mysterious. It is an extensive class of materials extending beyond the common window pane, however the only common factor between these different glasses is that they do not have anything in common in terms of their internal configurations, particularly in contrasted with exquisitely patterned and ordered crystals. The scale of glassy systems may range from metallic glasses consisting of atoms to sand castles consisting of grains of sand.
This steady critical strain offers insight into the basic seeds due to which failure in glasses occur – a small class of the constituent particles or atoms of glass that change shape collectively.
In spite of the disorder epitomizing glass, this combined activity seems to be universal among a number of glassy systems such as polymers, certain metals and colloids and is an essential starting point for the development of more durable materials.
Assistant professor Daniel Gianola of the Department of Materials Science and Engineering in Penn’s School of Engineering and Applied Science, graduate student Daniel Strickland together with graduate student Yun-Ru Huang and associate professor Daeyeon Lee of Engineering’s Department of Chemical and Biomolecular Engineering conducted the research. The findings were published in the Proceedings of the National Academy of Sciences.
Glasses can be classified as amorphous, meaning that the particles constituting them whether colloids, atoms or grains are configured such that there is no overarching pattern. The absence of internal consistency makes their behaviour difficult to foresee, particularly when it comes to how they fall apart or break. Crystalline materials with atoms lined up neatly in repetitive patterns may fail beginning at defects or at areas where the pattern is disordered.
These patterns are so periodic that researchers can envision the atomic structure of crystalline materials. However for their glassy counterparts, no such feature is available.
There are lots of ways to map out the position of atoms and defects in a crystal but we have no way of doing that for glasses. That’s why we need to build larger scale models if we’re going to understand how their atomic structure influences their behaviour.
Gianola
The model used by researchers in their study was developed at the lab of Daeyeon Lee. These were tiny pillars comprising microscopic plastic beads. Similar to wet sand, these beads could hold a shape because of capillary bridges that acted as a stand-in for atomic bonds.
We measure the stress at which the pillars deform permanently, and we found a surprisingly similar relationship between the way they behave and the way atomic disordered solids behave. In addition, the morphology of the failed pillars showed striking resemblance to atomic disordered solids.
Strickland
Shear bands are one among these resemblances, which are thin particle regions, which slide by their neighbours in most of the material while responding to stress. Though this is not prevalent in brittle oxide glasses such as the kind used in window panes, this is seen in a range of glassy materials from geological scales in earthquakes to atomic scales in metallic glasses.
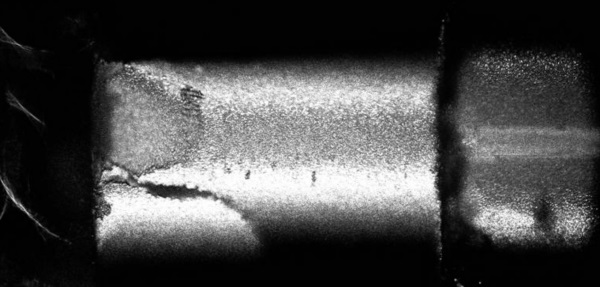
A microscope image of a micropillar failing after the formation of a shear band.
Among these resemblances are shear bands, thin regions of particles that slide by their neighbors in the bulk of the material in response to stress. Though they do not occur in brittle, oxide glasses like the kind used in window panes, this phenomenon is seen in a variety of glassy materials, from atomic scales in metallic glasses all the way up to geological scales in earthquakes.
In spite of its ubiquity, the mechanism behind shear banding and the critical strain value required to drive the phenomenon is least understood.
We want to better understand this kind of failure because we want to mitigate it if possible. If you’re engineering a good material, you don’t want that kind of failure to happen. It’s catastrophic and localized, when we’d rather it be graceful and uniform. You’d rather have a dent in your car bumper, rather than having the whole thing shear off.
Gianola
The researchers used a miniature piston to compress their micropillars, determining the force transmitted via them at the time of observing them when magnified. This test was run by tamping down the pillars to various densities. The researchers discovered that the material’s failure points with different stiffnesses falls on a fine line running through the glasses at a range of length scales.
Strickland stated that “At some level of applied stress, this fundamental event that leads to shear-banding becomes very favorable. We’ve shown that this point is roughly the same in atomic systems, polymer glasses, and in our micropillars.”
According to the findings it can be concluded these model systems are highly effective to study the “building blocks” of failure in glassy systems.
Gianola said “The slope of that line tells us about the fundamental unit of deformation in these glasses. It looks like it is associated with shape change in something like 10 to 100 particles, but ultimately leads to a system-spanning event, and it seems to be universal for all of these materials.”
“This is particularly surprising and interesting because it implies that plastic deformation is built on cooperative motion of the particles, in the case of our pillars, and atoms in atomic glasses,” he said. “So, despite the atomic randomness, there is some level of communication beyond single particles in a glass. This is in contrast to crystals, where any cooperative motion is trumped by individual defects.”
Developing this big-picture of this dissimilar material class signifies the interdisciplinary nature of Penn’s materials research team. The Gianola lab is an expert in hard materials and atomic solids and Lee’s group works with soft matter and colloids. Combining perspectives enables new insights, spanning a broad range of systems.
“Our research areas do not necessarily overlap on a first look,” Lee said, “but we were able to come up with a unique project through the support of Penn’s Materials Research Science and Engineering Center, which brings together groups with very different expertise. This project is a nice culmination of two seemingly disparate fields of research.”
Work being done by researchers uses in situ 3D confocal microscopy to track individual particles through the deformation process.
The National Science Foundation through Penn’s Materials Research Science and Engineering Center supported the research.
References