By Susha Cheriyedath, M.Sc.Reviewed by Skyla BailyFeb 21 2022
In a study published in the journal Materials, researchers evaluated the impact of microporosity on osteoconductivity and the osteoclastic resorbability of hydroxyapatite (HA)-based scaffolds for bone regeneration.
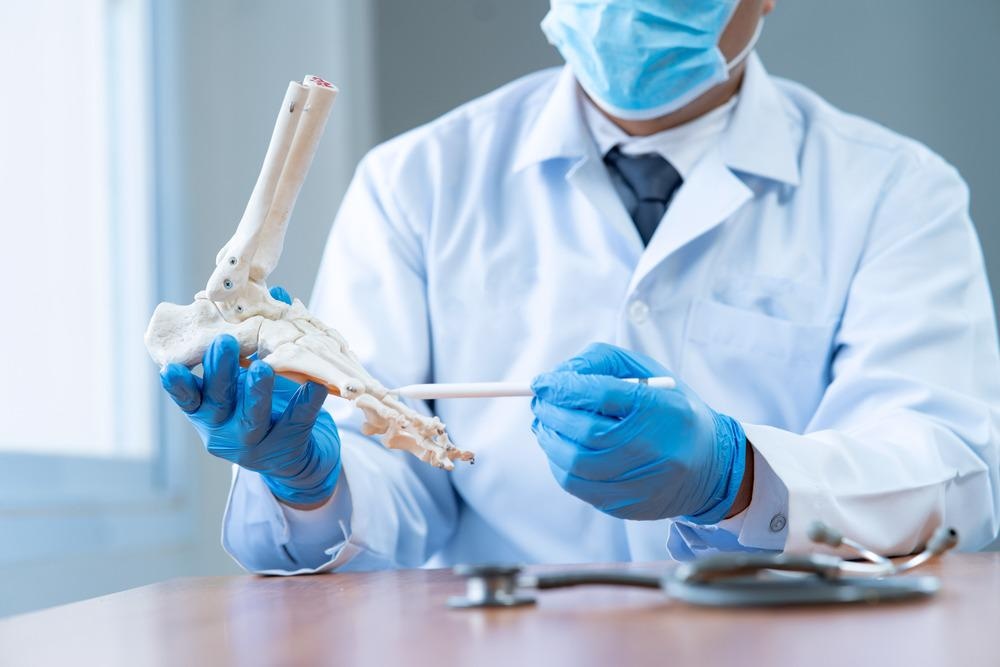
Study: 3D-Printed HA-Based Scaffolds for Bone Regeneration: Microporosity, Osteoconduction and Osteoclastic Resorption. Image Credit: Sirisak_baokaew/Shutterstock.com
Background
The need for user-friendly and convenient bone grafts has led to the emergence of bone tissue engineering, which aims at regenerating bone and bringing back its lost function in the body. The use of bone substitutes and scaffolds has been found to be advantageous for bone regeneration.
A method of building objects layer by layer called additive manufacturing is used for building bone substitutes. Three-dimensional (3D) printing with three levels of architecture can be used for manufacturing these substitutes. The micro- and macro-architecture of bone substitutes can be realized through additive manufacturing.
The bone defect determines the macroarchitecture while the implant is patient-specific and differs from the type of bone defect. The microarchitecture is defined by its predetermined distribution of 3D-printed material. The nanoarchitecture of 3D-printed scaffolds is influenced by post-processing techniques such as sintering temperature at a smaller scale. However, the relevance of scaffold microarchitecture and nanoarchitecture in osteoconduction remains unknown.
Macrographies and SEM images of the experimental scaffolds: (a) Scaffolds, which underwent different sintering temperatures, are shown to maintain their macro- and microarchitecture; (b) sintering temperatures applying to panel a and b are provided. SEM micrographs from the respective scaffolds are displayed with scales to the left; (c) the macroarchitecture of the full scaffold; (d) the macroarchitecture of the halved scaffold; (e) a picture taken after placement of all four different scaffolds in the calvarial bone defects of a rabbit is provided. Image Credit: Ghayor, C et al., Materials
Previous studies on tricalcium phosphate (TCP)-based scaffolds showed enhanced osteoconductivity at high microporosity. Since the bone is primarily made of HA crystals, researchers of the present study examined the use of HA scaffolds for bone regeneration. The research group also analyzed the properties of bone substitutes like osteoconduction, microporosity, and osteoclastic resorption.
About the Study
The team created lithography-based osteoconductive scaffolds using HA with equal macro- and microarchitecture but different nanoarchitecture. This was achieved by increasing the maximum sintering temperatures from 1100 to 1400 °C for changing microporosity. The microporosity, compression strength, and stiffness of the various scaffold types were also measured. In a calvarial defect model, the osteoconductivity of scaffolds was tested in vivo in 10 adult New Zealand White rabbits.
A scanning electron microscope (SEM) was used to analyze the scaffolds, while the microporosity was experimentally measured from the quantity of distilled water absorbed by the test scaffolds. A software program called TestXpert V11.02 was used to measure the maximal compression strength, while bony bridging was determined as a measure of osteoconduction.
![Comparison of osteoconductivity in relation to microporosity and compression strength between otherwise identical HA- and TCP-based scaffolds. The results for TCP-based scaffolds produced with the identical stl-file were generated and reported earlier [10]. (a) Osteoconductivity related to microporosity of test samples; (b) osteoconductivity related to compression strength of the partially sintered scaffolds. The values are displayed as mean ± standard deviation. The range for cancellous bone (2–12 N/mm2) depicted in the green shaded area was taken from [34].](https://www.azom.com/images/news/ImageForNews_58284_1645438235038609.jpg)
Comparison of osteoconductivity in relation to microporosity and compression strength between otherwise identical HA- and TCP-based scaffolds. The results for TCP-based scaffolds produced with the identical stl-file were generated. (a) Osteoconductivity related to microporosity of test samples; (b) osteoconductivity related to compression strength of the partially sintered scaffolds. The values are displayed as mean ± standard deviation. Image Credit: Ghayor, C et al., Materials
Observations
The HA-based scaffolds were analyzed to examine whether microporosity influenced the formation of bone, osteoconduction, and osteoclastic resorption. The team inferred that the osteoconduction and recovery of a cranial deformity using scaffolds of indistinguishable and open permeable microarchitecture was dominant for all platform types.
Also, no critical contrast between top sintering temperatures from 1100 to 1400 °C and microporosities in the range of 0 and 46% has been distinguished. The HA scaffolds showed higher osteoconductivity compared to TCP-based ones, and on the periphery of these HA scaffolds, the formation and disintegration of osteoclasts were observed.
HA grains grew based on the sintering temperature from 1.07 ± 0.46 µm at 1100 °C to 5.98 ± 1.11 µm at 1400 °C and also underwent partial fusion. The diameter of the micropore expanded from 1.17 ± 0.47 µm at 1100 °C to 1.67 ± 0.52 µm at 1400 °C. In addition, surface and microporosity were impacted by partial sintering. While the surface reduced from 0.79 m2/g at 1100 °C to 0.24 m2/g at 1400 °C and microporosity decreased from 45.85 ± 0.39% to 0.74 ± 1.87%.
By adjusting the sintering temperature, the researchers were able to control grain size, micropore diameter, and overall microporosity. Microporosity was analyzed from the weight gain caused due to infiltration with distilled water. HA-based scaffolds were adequate to improve the in vivo bone formation since they exhibited an increase in microporosity from 10 to 20% for a porosity of 80%.
High osteoconductivity was observed with sintering temperatures between 1100 and 1300 °C and microporosities at 18 and 46%. Since osteoconductivity decreased at 1400 °C, phase transformation had a minor impact on osteoconductivity or osteoclastic degradability.
The HA scaffold was found to be better compared to TCP for the production of wide-open scaffolds with high mechanical strength and osteoconductivity. The Ca2+ dissolution curves of the HA-based scaffolds appeared parallel and were similar for all sintering temperatures. The team concentrated on HA-based scaffolds with grain sizes in the range of 1.07 and 5.98 µm and witnessed osteoclast arrangement and movement on every surface and grain size.
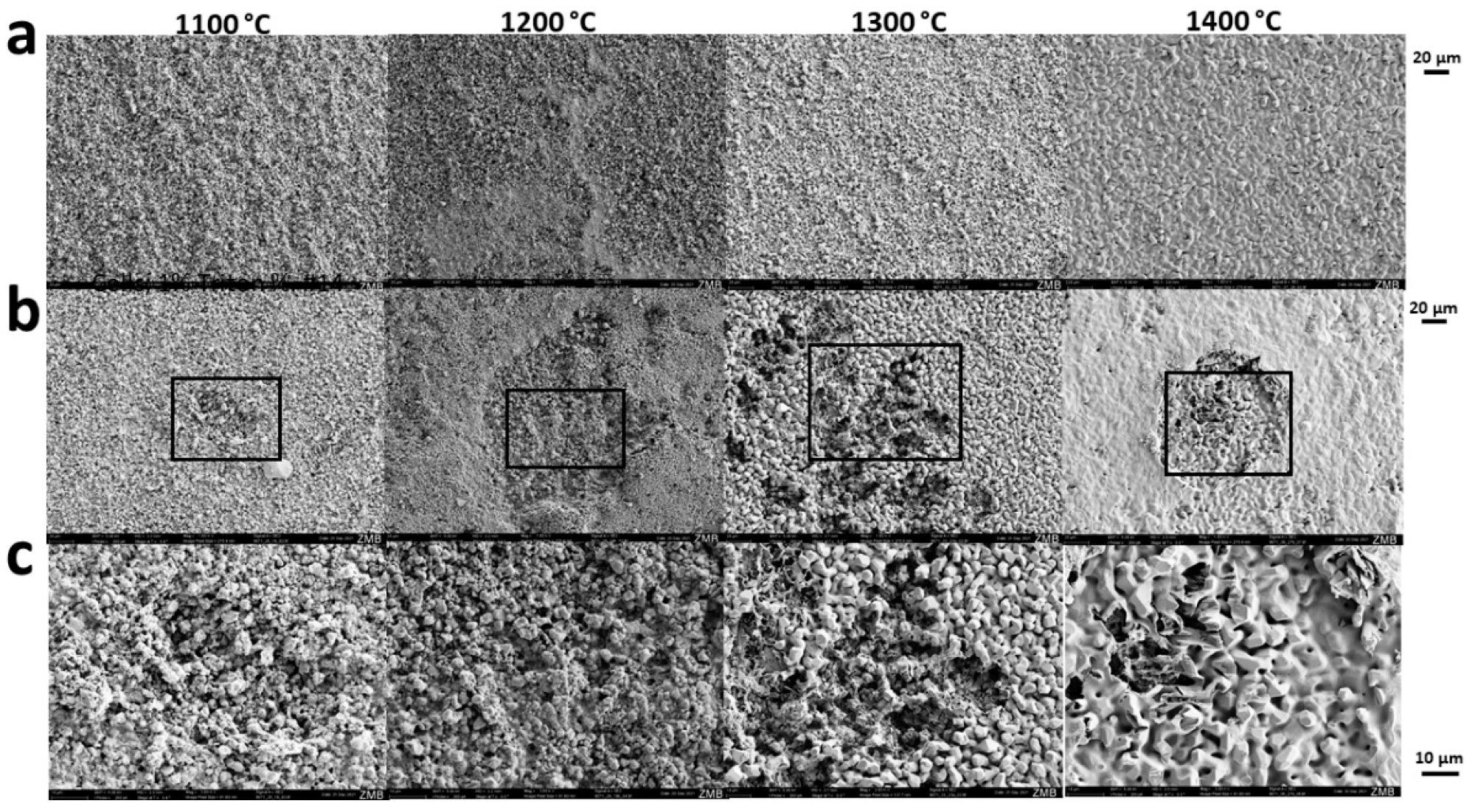
Sintering-temperature dependent osteoclastic degradation of scaffolds. Scanning electron microscopy was performed from scaffolds not exposed to cells (a) or seeded and exposed to osteoclastic RANKL-stimulated RAW264 cells (b,c). The maximal sintering temperature is provided at the top of each column. Scales of each panel are provided. Image Credit: Ghayor, C et al., Materials
Conclusions
The positive impact of microporosity on osteoconduction in TCP-based scaffolds prompted the researchers to study the impact of microporosity on osteoconduction in HA-based scaffolds. The results showed that HA-based scaffolds had high osteoconductivity without depending on the microporosity. Also, they were considered more qualified for bone recovery methods.
Besides, microporosity somewhere in the range of 0 and 46% had no significant impact on osteoclast arrangement and osteoclastic resorbability. Hence, according to the researchers, microporosity had not influenced osteoconductivity or osteoclastic resorbability. These discoveries pave the way for future improvements in 3D-printed calcium phosphate-based scaffolds.
Disclaimer: The views expressed here are those of the author expressed in their private capacity and do not necessarily represent the views of AZoM.com Limited T/A AZoNetwork the owner and operator of this website. This disclaimer forms part of the Terms and conditions of use of this website.
Source:
Ghayor, C.; Bhattacharya, I.; Guerrero, J.; Özcan, M.; Weber, F.E. 3D-Printed HA-Based Scaffolds for Bone Regeneration: Microporosity, Osteoconduction and Osteoclastic Resorption. Materials 2022, 15, 1433. https://www.mdpi.com/1996-1944/15/4/1433