By Surbhi JainReviewed by Susha Cheriyedath, M.Sc.Mar 17 2022
In an article recently published in the journal ACS Energy Letters, researchers discussed the mechanism of the photocatalytic hydrogen production reaction at the interfaces of water and tin halide perovskite.
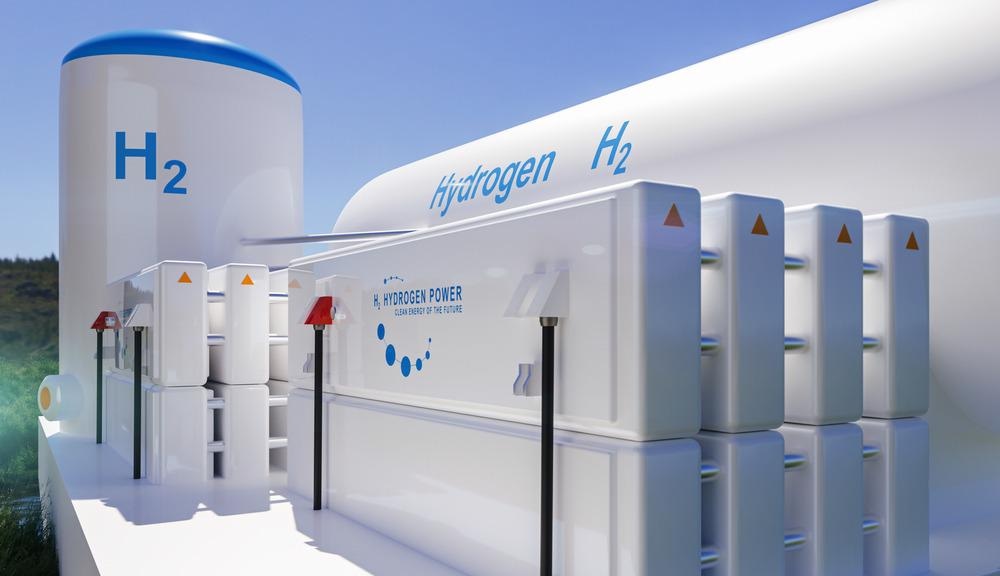
Study: Reaction Mechanism of Photocatalytic Hydrogen Production at Water/Tin Halide Perovskite Interfaces. Image Credit: Audio und werbung/Shutterstock.com
Background
Due to the long lifetime of photogenerated carriers, high absorption coefficients, and fault tolerance, metal halide perovskites are interesting materials for photovoltaics. Lead-based materials, in particular, have risen quickly as competitors to silicon in the photovoltaics landscape due to these features.
The replacement of lead with tin is a viable option for reducing the potential toxicity of lead-containing items. Although tin halide perovskites (THPs) have a lower bandgap, the efficiency and stability of the resulting solar cells are limited. Owing to their band edge tunability, THPs are also intriguing as photocatalysts in theory.
While the inability of metal halide perovskites to function in aqueous environments has long been a barrier to their use in heterogeneous photocatalysis, new research has demonstrated that some THPs can be water-stable and active in photocatalytic hydrogen production.
Metal halide perovskites, while potentially appropriate for photocatalysis, are frequently unstable when exposed to moisture and water, restricting their use in watery environments. This paradigm has lately evolved as new perovskites that are both water-stable and lead-free have been reported. Bi- and Sn-based perovskites have shown promising results, demonstrating both water stability and good photocatalytic capabilities.
About the Study
In the present study, the authors discussed the utility of advanced electronic structure calculations to compare the reactivity of the active and water-stable DMASnBr3 (DMA = dimethylammonium) perovskite against prototypical MASnBr3 and MASnI3 compounds (MA = methylammonium) to unravel the mechanistic details underlying the photocatalytic activity of THPs.
The conduction band energetics, which drives the binding energy of electron polarons at the surface of THPs, were discovered.
The team illustrated the impact of the interaction between the A-site cation and the halogen on the material's photo-reactivity in terms of the regulation of the perovskite electronic energy levels. More stable and efficient photocatalytic hydrogen reduction materials were developed by explaining the key phases of the reaction.
The researchers compared the archetypal tin perovskites MASnI3 and MASnBr3 with the water-stable DMASnBr3 using high-level density functional theory calculations. They evaluated the electronic structure of the proposed materials, and for MASnI3 and DMASnBr3, the binding energies of defects and polarons for various slabs were determined.
More from AZoM: Using X-Ray Fluorescence for Trace Element Identification in Food
The interaction of the perovskite with water was simulated, and both AX- and SnX2-terminated surfaces were used to reconstruct the overall hydrogen generation reactivity pattern. The electron-trapping and photocatalytic activity of THPs were also examined using the archetypal MASnI3 and the recently synthesized water-stable and photoreactive DMASnBr3.
Observations
The density of states (DOS) demonstrated that the interplay of the halides and the A-site cations contributed to a significant tuning of the material band edges. As a consequence of the valence band (VB) downshift of 0.8 eV and conduction band (CB) upshift of 1.2 eV, the predicted bandgap increased from 1.1 eV in MASnI3 to 3.2 eV in DMASnBr3. When a second electron was added, charge localization was found to be linked to the creation of Sn-Sn dimers with bond lengths ranging from 3.1 to 3.3Å. For all models investigated, water dissociation was significantly disfavored, with ΔE0diss (H2O) > 1.5 eV.
The presence of electron polarons at the surface of THPs, whose binding energy was directly connected to the position of the conduction band edge, played an important role in the photocatalytic hydrogen reduction activity. The comparative analysis revealed that the conduction band energetics-determined binding energy of the surface electron polarons played a dual role in the photocatalytic production of hydrogen. Along with providing the primary driving force for the reaction, the trapping obstructed the electron transfer toward hydrogen reduction.
The results also showed that the interaction between the cation and the halide was a critical factor that influenced the stability and photocatalytic effectiveness of THPs.
Conclusions
In conclusion, this study elucidated that by tweaking the perovskite electronic energy levels, the material’s chemical composition, in terms of halogen and A-site cation, plays a vital role in defining the material's photo-reactivity. The authors emphasized that the results of this study could aid in the development of more efficient and stable photocatalytic hydrogen reduction materials by explaining the crucial processes in the interfacial photocoupled electron transfer mechanism.
They also believe that because the photo-reactivity of THPs is determined by the location of their electronic energy levels, the interaction between the A-site cation and the halogen in modifying this feature can be used to generate more stable and efficient photocatalytic hydrogen reduction materials.
Disclaimer: The views expressed here are those of the author expressed in their private capacity and do not necessarily represent the views of AZoM.com Limited T/A AZoNetwork the owner and operator of this website. This disclaimer forms part of the Terms and conditions of use of this website.
Source:
Ricciarelli, D., Kaiser, W., Mosconi, E., et al. Reaction Mechanism of Photocatalytic Hydrogen Production at Water/Tin Halide Perovskite Interfaces. ACS Energy Letters 1308-1315 (2022). https://pubs.acs.org/doi/10.1021/acsenergylett.2c00122