A group of researchers recently published a paper in the journal Nature Communications that demonstrated the creation of two-dimensional (2D) solid helium using the diamond lattice confinement effect.
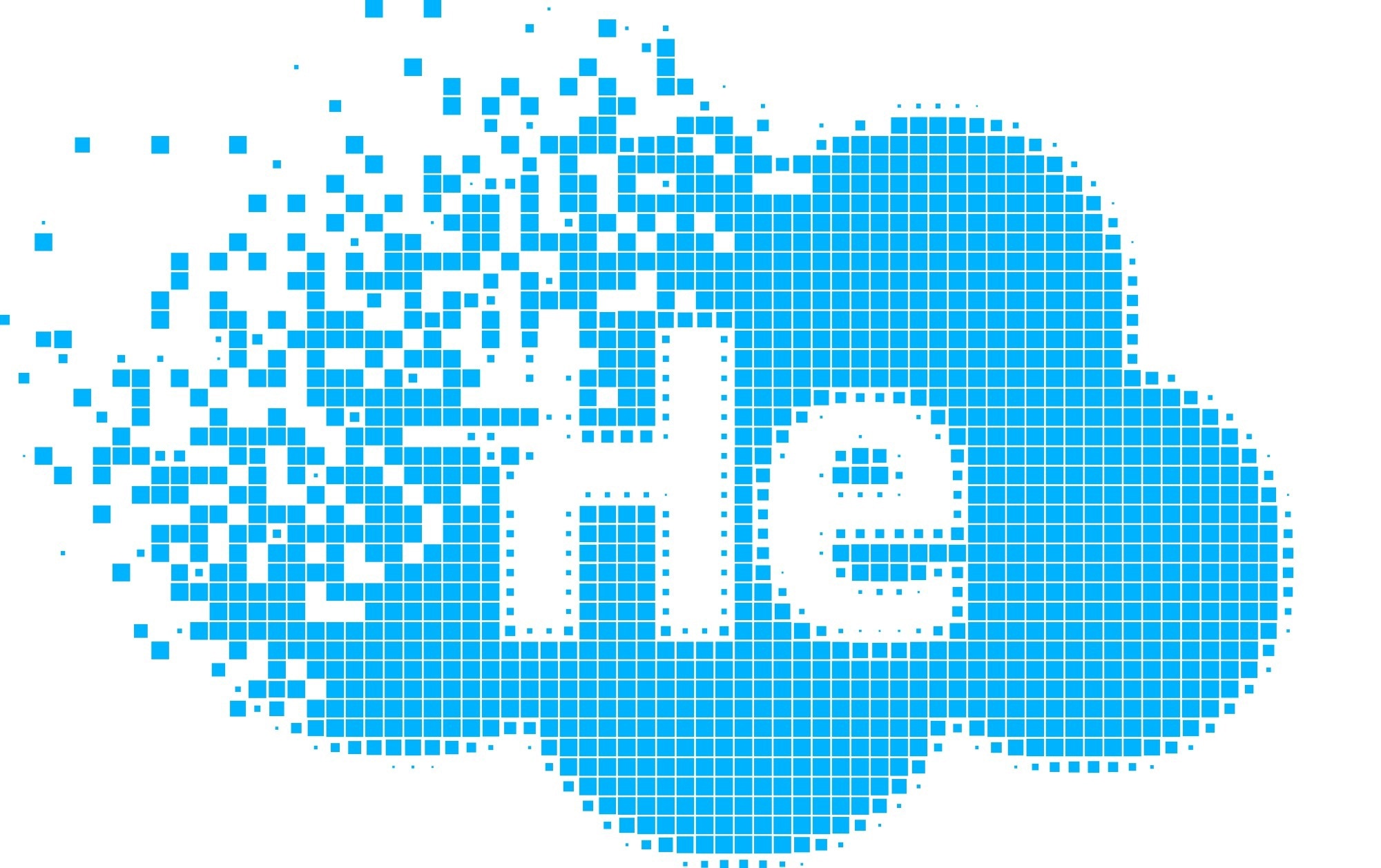
Study: Creating two-dimensional solid helium via diamond lattice confinement. Image Credit: Digital Clipart/Shutterstock.com
Background
Solid helium is found extensively in the universe in polymorphic form. Solid helium forms when helium transforms into typical quantum crystals with exceptional physical properties, such as giant plasticity, superfluidity, and second sound at low temperatures. Exotic states of matter and quantum atomic physics can be explored by stabilizing solid helium in two dimensions.
However, the production, observation, and utilization of solid helium are considerably challenging as high-pressure techniques are needed to solidify it. Helium typically solidifies at more than 11.5 GPa pressure at room temperature due to its quantum crystalline nature.
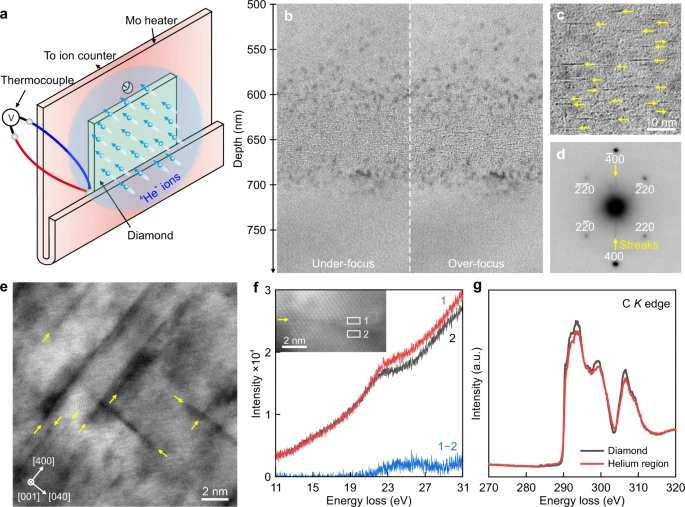
High-temperature 4He+ ion implantation. a Schematic of 4He+ ion implantation into diamond at high temperature. b Fresnel contrast TEM images show helium ion implantation region in the under-focus (Δf = −400 nm) and over-focus (Δf = +400 nm) conditions. c Higher-magnification Fresnel contrast TEM image demonstrates helium platelets, which are highlighted by yellow arrows, in the over-focus (Δf = +200 nm) condition. A two-beam condition g = 400 was used. d Selected area electron diffraction pattern of the helium region confirms the formation of {100} helium platelets. e HAADF STEM image illustrates {100} helium platelets. f Low-loss electron energy-loss spectra obtained on (area 1) and off (area 2) the platelet. g Core-loss electron energy-loss spectra show carbon K edge from the helium ion implantation region and the pristine diamond. Image Credit: Lin, W et al., Nature Communications
Synchrotron X-ray techniques have been previously used with diamond anvil cells to investigate the bulk solid helium and helium compound structures. Moreover, 2D solid helium adsorbed on the graphite surface was evaluated extensively below 7.4 K as a simple model to understand phase transitions. However, direct manipulation and observation of 2D solid helium at room temperature have not been performed until now due to the lack of suitable high-pressure devices.
2D solid helium specimens can be fossilized through the lattice confinement effect, as helium is insoluble in solids. This concept of trapping helium at room temperature in a condensed state has been evaluated for helium-implanted metals. Additionally, helium confined in silicon was also investigated extensively, and helium nanobubbles were found suitable for silicon technology.
Implanted helium ions precipitate spontaneously into nanoscale helium platelets when the available vacancies are negligible in the lattice where the helium atoms are confined in a 2D structure. The surrounding crystal lattice generates high static pressures, which can stabilize the 2D solid helium at ambient temperature.
Diamond, the hardest material on earth, is considered most suitable to achieve 2D solid helium as diamond lattice can exert sufficient pressure on helium. However, achieving 2D solid helium controllably through diamond lattice confinement is difficult as suppressing the radiation-induced graphitization and amorphization of the lattice during the implantation of helium ions is extremely challenging.
Such irradiation damage-induced structural instability can significantly reduce the diamond stiffness, leading to considerably lower pressure within platelets or preferential helium nanobubble formation. Moreover, helium diffusion remains substantially limited within the diamond lattice due to the strong and short sp3-bonded carbon structure, which inhibits helium platelet formation.
The Study
In this study, researchers created 2D solid helium at room temperature through the diamond lattice confinement effect. 275 keV 4He+ ions were implanted in diamonds at 1573 K using a high-voltage Engineering Europe 500 kV ion implanter. Diamonds were placed in a vacuum chamber with pressure less than 10−6 torr during implantation.
Platinum/platinum-rhodium (Pt/Pt-Rh) thermocouples were used to precisely control the sample temperature based on the active temperature feedback. Helium concentration and irradiation damage along the cross-section direction were predicted by the stopping and range of ions in matter code with the quick Kinchin-Pease mode.
Researchers performed atomic-resolution scanning transmission electron microscopy (STEM), electron energy loss spectroscopy (EELS), high-resolution TEM imaging, high-angle annular dark-field imaging (HAADF) STEM imaging, atomic-resolution integrated differential phase contrast microscopy (iDPC) STEM imaging, selected area electron diffraction, and Fresnel contrast imaging for microstructural characterization.
Researchers also developed an atomic structural model of the samples. The crystal structure of 2D solid helium was constructed by inserting the helium atomic layers into the cubic diamond.
Three different interstitial helium sites in the diamond lattice and two-different in-plane atomic ratios were considered and calculated. The effect of helium interlayer spacing on cell pressure at the computing limits was also investigated. Atomic internal freedoms were optimized for other structural models.
All lattice parameters and atomic positions were fully relaxed only for the initial diamond to modulate the confined helium platelet in the diamond lattice using density functional theory (DFT) calculations.
![Evidence of two-dimensional solid helium confined by diamond. a iDPC STEM images (upper) and the corresponding simulated atomic structure (lower) of the pristine diamond viewed along the [011] zone axis. iDPC STEM images (upper) and the corresponding simulated atomic structures (lower) of diamonds containing two-dimensional solid helium viewed from (b) [011], (c) [01¯101¯1] and (d) [001] zone axes. Blue arrows highlight helium platelets confined by the diamond lattice. The top and bottom diamond lattices are included in the simulated atomic structures.](https://d12oja0ew7x0i8.cloudfront.net/images/news/ImageForNews_60225_1665651177953521.png)
Evidence of two-dimensional solid helium confined by diamond. a iDPC STEM images (upper) and the corresponding simulated atomic structure (lower) of the pristine diamond viewed along the [011] zone axis. iDPC STEM images (upper) and the corresponding simulated atomic structures (lower) of diamonds containing two-dimensional solid helium viewed from (b) [011], (c) [011] and (d) [001] zone axes. Blue arrows highlight helium platelets confined by the diamond lattice. The top and bottom diamond lattices are included in the simulated atomic structures. Image Credit: Lin, W et al., Nature Communications
Observations
The 2D solid helium atomic structure was visualized and manipulated controllably at ambient temperature due to the use of the robust diamond lattice. The issues associated with the diamond lattice confinement of helium ions were addressed by increasing the helium ion implantation temperature to 1573 K, which simultaneously improved the helium diffusion rate and point defect recombination in the diamond lattice.
The 2D solid helium led to unique diamond lattice strain engineering responses, which can facilitate the development of diamond devices with tunable bandgap characters and potentially exceptional functional properties.
Specifically, the high-pressure 2D solid helium atoms generated significant elastic strain on the diamond lattice. The 2D solid helium monolayer-induced compressive strains to the surrounded diamond lattice narrowed the large bandgap of the diamond by 2.2 eV.
This strain doping effect can substantially change the inherent electronic features of the diamond, making it suitable for unique applications in semiconductor electronics. Thus, deep elastic engineering of diamonds can be achieved reliably by implanting 2D solid helium.
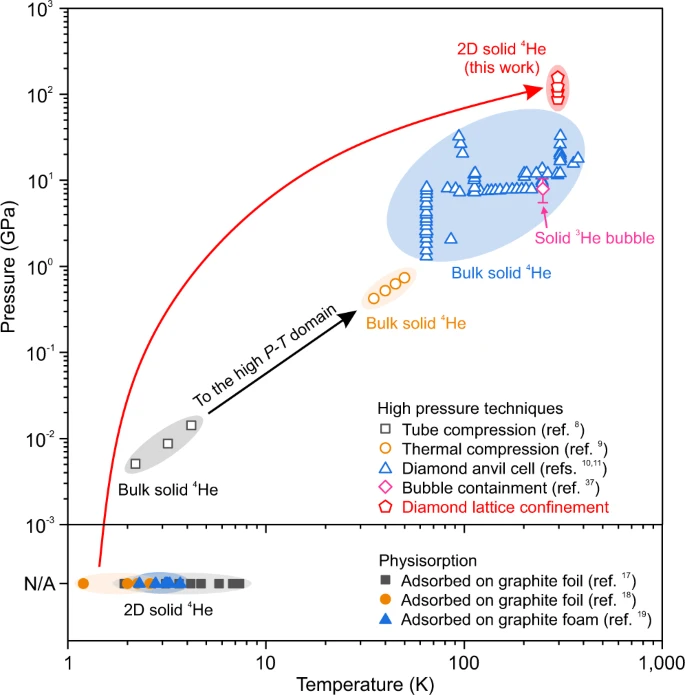
P-T domain of three-and two-dimensional solid helium. Helium was first solidified by Keesom8 in 1926. With the development of high-pressure techniques9,10,11,37, three-dimensional solid helium has been obtained at high P-T conditions. Nevertheless, two-dimensional solid helium has previously been achieved by physisorption on the surface of graphite at low temperatures17,18,19. Using diamond lattice confinement, we substantially extended the P-T domain of two-dimensional solid helium for potential practical applications. Image Credit: Lin, W et al., Nature Communications
The 2D solid helium possessed 0.315 atom/Å2 areal density. The helium platelets confined in the diamond lattice demonstrated a 2D and atomically thin structure, with a 4.6 nm mean diameter and 0.24 nm average opening, and robust anisotropic distribution.
Controllable ion implantation enabled the self-assembly of monolayer helium atoms between {100} diamond lattice planes. The helium interlayer pressure increased with the decreasing platelet spacing. Solid helium monolayers displayed an anisotropic nature with a buckled tetragonal arrangement.
The average interlayer spacing was 3.0 ± 1.3 nm between {100} helium platelets with a one nm minimum platelet spacing when the maximum helium pressure was 166 GPa, indicating a strong lattice confinement effect that facilitated the successful formation of 2D solid helium.
To summarize, the findings of this study demonstrated that the synthesis of 2D solid helium through the diamond lattice confinement effect could allow steerable manipulation of solid helium to achieve intrinsic strain doping with profound applications.
Disclaimer: The views expressed here are those of the author expressed in their private capacity and do not necessarily represent the views of AZoM.com Limited T/A AZoNetwork the owner and operator of this website. This disclaimer forms part of the Terms and conditions of use of this website.
Source:
Lin, W., Li, Y., de Graaf, S., et al. Creating two-dimensional solid helium via diamond lattice confinement. Nature Communications 2022. https://www.nature.com/articles/s41467-022-33601-5