Scientists from Fudan University in China have provided new insights into semiconducting polymer thermoelectric materials by leveraging chemical doping and molecular structure modification strategies. Their findings have been published in the journal ACS Energy Letters.
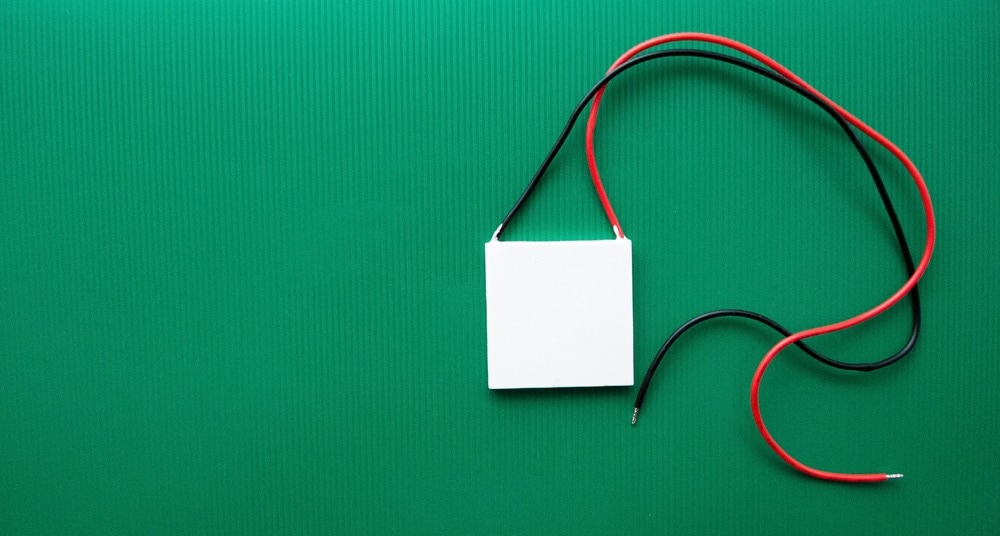
Study: Strategic Insights into Semiconducting Polymer Thermoelectrics by Leveraging Molecular Structures and Chemical Doping Image Credit: Axelvidal/Shutterstock.com
Thermoelectric Energy Conversion
The growing evidence for climate change has focused the attention of researchers on new environmentally friendly low-carbon emission technologies to reduce the ecological impact of fossil fuel use.
One of the most promising fields of research is thermoelectric energy conversion. These devices can directly transform heat into electricity and operate on the principle of the Peltier and Seebeck effects.
Amongst the various materials evaluated as thermoelectrics, inorganic semiconductors have been extensively researched, with favorable properties demonstrated in studies. However, inorganic semiconductors display some crucial drawbacks.
Organic Semiconducting Thermoelectric Polymers
Semiconducting polymers have become a focus of study over the past few years in areas such as organic photovoltaics, field-effect transistors, and LEDs. These materials possess some key benefits, such as mechanical flexibility, processability at low temperatures in solution, and easy tunability of mechanical and optical properties.
These materials have merits compared to their inorganic cousins, such as low thermal conductivity, non-toxicity, and good mechanical deformability performance. Organic polymers also have the benefits of enhanced mechanical, thermal, chemical, and irradiation stability compared to small molecules. Their superior bendability allows them to adhere to thermal surfaces effectively.
These materials display vast potential for use in applications such as wearable electronics. Chemical doping can improve the electrical properties, such as conductivity, of organic semiconducting polymers. However, these materials possess poor doping efficiency, which limits their performance compared to their inorganic counterparts.
The Paper
The new paper in the journal ACS Energy Letters is a focused review of the current advances in molecular tailoring and chemical doping strategies for organic semiconductor polymer thermoelectrics.
The authors have critically unraveled the electrical doping mechanisms in these materials and have comparatively discussed molecular dopants. Types of dopants are compared according to their energy level alignment and underlying mechanisms. By comparing these factors, the authors have presented numerous suitable n-type and p-type dopants and chemical doping enhancement strategies.
Design strategies are discussed in depth in the paper, with an aim to improve doping efficiencies. Several novel strategies are proposed to improve various electrical properties and parameters in this class of materials.
Additionally, novel strategies have been investigated by the authors for improving film morphologies that decouple the Seebeck coefficient and electrical conductivity. Finally, the main roadblocks which hinder the future development of high-performance organic semiconducting polymer thermoelectrics are presented by the authors alongside strategies to overcome them.
Chemical Doping, Dopant Types, and Strategies
The paper discusses two main factors which impact the thermoelectric performance and charge carrier transport performance of semiconducting polymers. These are the choice of dopant and the dopant’s underlying mechanisms, and the doping strategies. Chemical dopants significantly influence the microscopic morphologies and molecular packing behaviors of polymers.
Types of dopants highlighted in the paper include commonly exploited p-type dopants such as TCNQ derivatives and n-type dopants such as CoCp2. Other dopants include N-DMBI and p-type dopants such as FeCl3, a strong ferric ion salt oxidant. Dopant positions in the polymer film’s amorphous or crystalline regions can influence behaviors such as free carrier release.
Several studies have focused on the optimization of dopants and doping strategies. Chemical doping can be separated into two types: acid-base doping and charge-transfer doping. Additionally, there are various doping strategies including sequential doping, mixed doping, and other emerging methods, each with its own unique advantages and disadvantages.
Correlating the Structure of Polymers and Their Electrical Properties and Supplementary Strategies
An in-depth investigation of how polymer structure relates to electrical characteristics has been presented by the authors. Due to this intimate correlation, strategies which modulate polymer side chains and the π-backbone are highly effective at optimizing the electrical performance of these materials.
Multiple studies have investigated novel approaches to achieve these performance enhancements. Strategies that enhance free carrier release have also been evaluated in recent research. Supplementary strategies including polymer alignment approaches, mechanical stretching, and developing polymer blends have been explored to enhance the thermoelectric performance of materials.
Future Outlook on Strategies
Chemically doped organic semiconducting polymers display superior bendability to other currently explored materials and hold notable potential for use in thermoelectric applications for wearable electronics and other key innovative research areas. There is much room for improving their thermoelectric performance currently.
A number of potential research directions which can enhance the performance of these materials have been presented in the focus review. These include designing p-type D-A polymers which can take advantage of the relatively larger energetic disorder in their backbones.
Chemical doping levels should be finely tuned to provide a balance between different parameters. Enhancing free carrier release during doping should be investigated further. Strategies to enhance carrier mobility and electrical conductivity using different materials, dopants, and doping approaches should be investigated by future researchers.
Other key areas of future research should include clarifying the effect of dopants on thermal conductivity and enhancing the stability of doped polymers. Novel dopants and emerging polymers should be explored extensively. Whilst this remains a highly challenging area of materials science, the opportunities for manufacturing high-performance semiconducting polymer thermoelectrics are many.
More from AZoM: How are Bioplastics Made?
Further Reading
Tang, J et al. (2022) Strategic Insights into Semiconducting Polymer Thermoelectrics by Leveraging Molecular Structures and Chemical Doping ACS Energy Letters 7 pp. 4299-4234 [online] pubs.acs.org. Available at:
Disclaimer: The views expressed here are those of the author expressed in their private capacity and do not necessarily represent the views of AZoM.com Limited T/A AZoNetwork the owner and operator of this website. This disclaimer forms part of the Terms and conditions of use of this website.