A team of researchers recently published a paper in the journal SusMat that demonstrated the feasibility of using a sodium-gallium alloy layer to prevent dendrite growth on sodium metal anodes to improve the stability and electrochemical performance of sodium metal batteries.
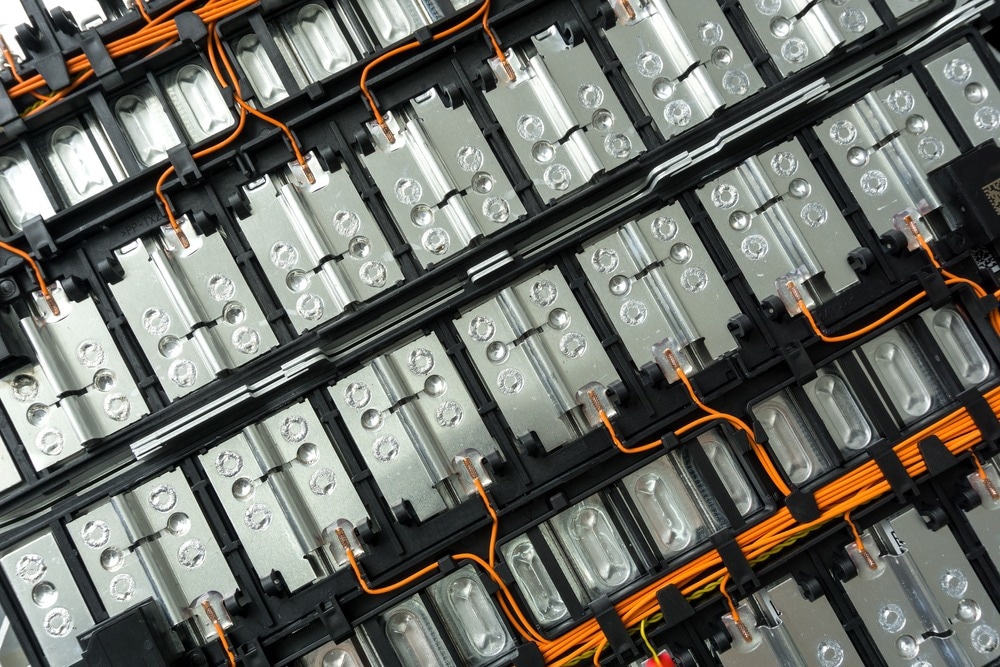
Study: Sodium–gallium alloy layer for fast and reversible sodium deposition. Image Credit: Smile Fight/Shutterstock.com
Background
The rising demand for power in portable electronics, smart grids, and electric vehicles has considerably increased the importance of high-energy batteries. In previous decades, lithium-based batteries, such as lithium-metal batteries (LMBs), have gained significant attention as energy storage.
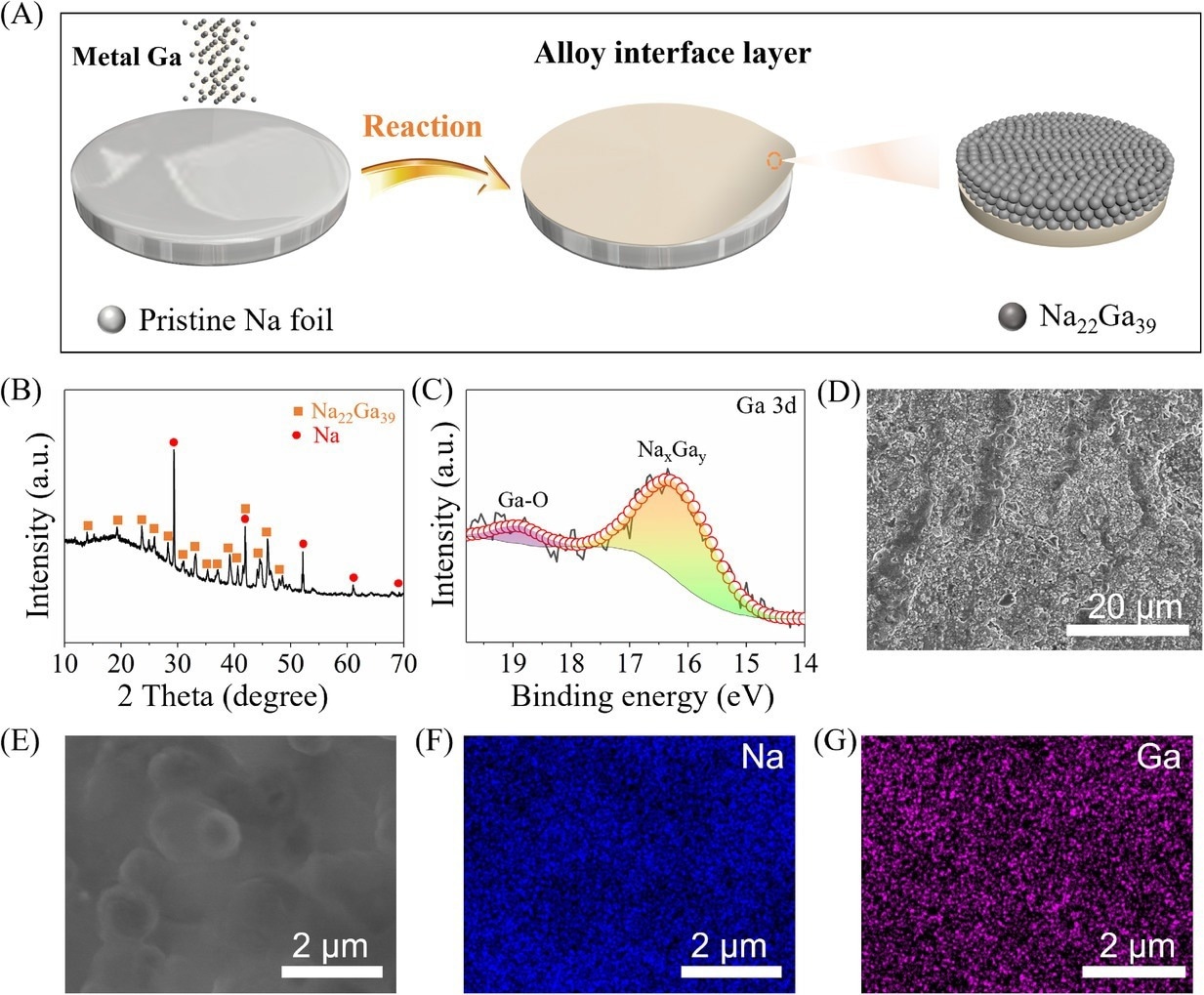
(A) Schematic illustration of the fabrication process of Na–Ga alloy layer by rolling the metal Ga on Na metal (NGAL-Na). Characterization of NGAL-Na: (B) X-ray diffraction (XRD) pattern, (C) the deconvoluted X-ray photoelectron spectrometry (XPS) spectrum of Ga 3d, (D) scanning electron microscopy (SEM) image, and (E–G) elemental mapping of Na and Ga. Image Credit: Lv, X et al., SusMat
LMBs, including lithium-oxygen and lithium-sulfide batteries, possess a considerably higher energy density. However, low lithium metal reserves in the world can hinder the development of these batteries.
Sodium metal batteries with similar chemical properties as LMBs are considered a suitable alternative as sodium metal is abundant in nature and possesses a high theoretical specific capacity of 1166 mAh g-1 and low redox potential.
However, safety issues and poor cyclic stability must be addressed to obtain a stable sodium metal anode. Sodium metal spontaneously forms a solid electrolyte interphase (SEI) when it comes in contact with organic liquid electrolytes.
Inhomogeneous sodium ion flux striping/plating can lead to sodium dendrite propagation, damage of unstable SEI, and depletion of electrolytes. Sodium dendrite propagation can increase the interfacial instability of sodium metal, hindering its practical implementation.
Collectively, these factors can shorten the lifetime of the sodium anode. Several strategies, such as using solid-state electrolytes (SSEs), introducing three-dimensional (3D) porous collectors, and using additives into electrolytes, were implemented to prevent sodium dendrite growth and improve sodium anode stability.
However, these strategies have significant drawbacks. For instance, the 3D porous collector introduction cannot prevent the parasitic reactions with the electrolyte, while additives do not last for long cycles. Fabricating different artificial interphase layers is considered an effective approach to suppressing sodium dendrite growth.
Although polymers and metal chalcogenides have been used as protective layers on sodium metal, the expansion of volume during the long stripping/plating cycles typically breaks or destroys these protective layers from the metal, which necessitates the development of new protective layer types.
Alloy layers can promote interfacial dynamics and fast charge transport. Additionally, alloy layers can reduce the parasitic reactions with organic liquid electrolytes due to their higher inertness than pure metals. Thus, using alloy layers can lead to a flat morphology for metal ions and uniform plating with no dendrite growth.
Gallium with a low melt temperature of 30 oC can be used as an alloy layer for sodium metal. However, no study has used the sodium-gallium alloy layer to improve the sodium anode interface stability until now.
The Study
In this study, researchers fabricated a sodium-gallium alloy layer through in-situ rolling of gallium metal on the sodium metal surface (NGAL-Na) and evaluated its ability to improve the sodium anode interface stability by preventing dendrite growth.
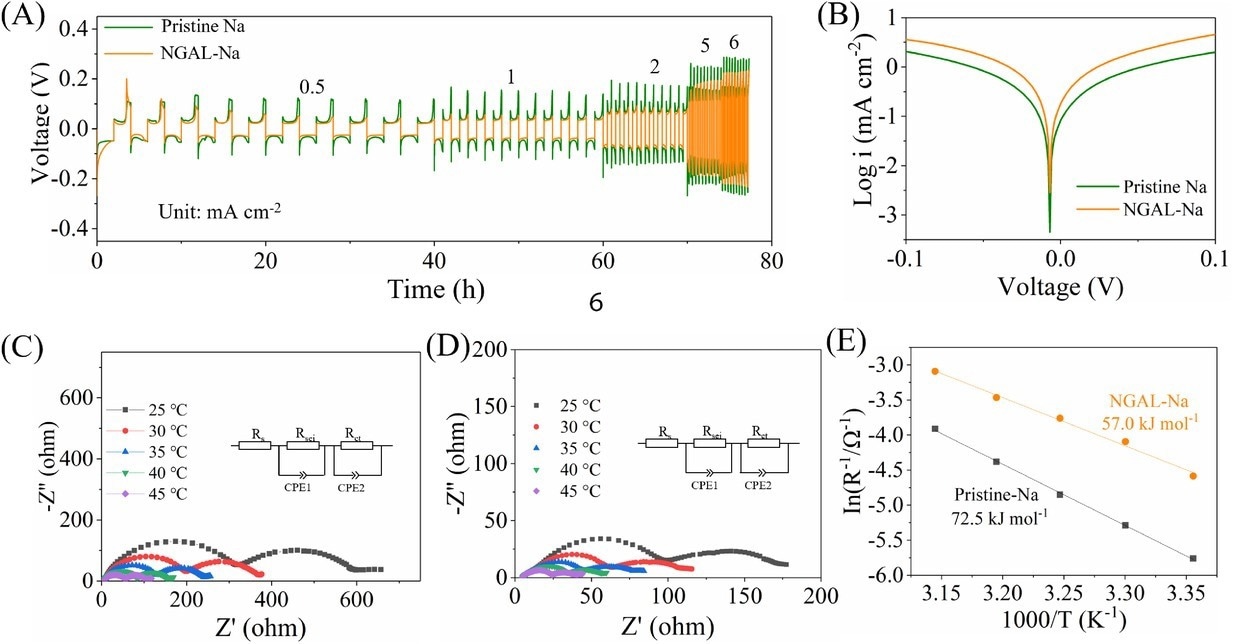
(A) Rate performance at current densities of 0.5–6 mA cm−2 (setting capacity: 1 mA h cm−2). (B) Tafel plots. Nyquist plot at various temperatures and equivalent circuit model for (C) bare Na and (D) Na–Ga alloy layer by rolling the metal Ga on Na metal (NGAL-Na). (E) Arrhenius behavior of symmetric Na||Na and NGAL-Na|| NGAL-Na cells. Image Credit: Lv, X et al., SusMat
X-ray diffraction, X-ray photoelectron spectroscopy, and field emission scanning electron microscopy were used to characterize the fabricated samples. The fabricated NGAL-Na anode was punched into 11 mm discs for coin-cell testing.
Symmetrical CR2032 coin-type cells were used to perform the electrochemical test for symmetric NGAL-Na||NGAL-Na and sodium||sodium cells using one M sodium perchlorate in ethyl carbonate/diethyl carbonate (EC/DEC) with 5% fluoroethylene carbonate (FEC) as electrolyte.
The Whatman GF/D glass fiber was utilized as a separator in all batteries. Full cells were investigated by coupling with commercial sodium vanadium phosphate Na3V2(PO4)3/(NVP) electrode. An electrochemical workstation was used to perform the electrochemical impedance spectroscopy (EIS).
Rate performance and long-term cyclic stability of symmetric cells were performed using a Neware testing instrument. Additionally, transparent quartz cells were assembled to observe the sodium deposition surface microscopy for NGAL-Na and bare sodium anodes.
Significance of the Study
The NGAL-Na anode was fabricated successfully through gallium metal rolling on a bare sodium surface at room temperature. The sodium-gallium alloy layer with a well-defined Na22Ga39 composition was developed after the in-situ alloying reactions between sodium and gallium during rolling.
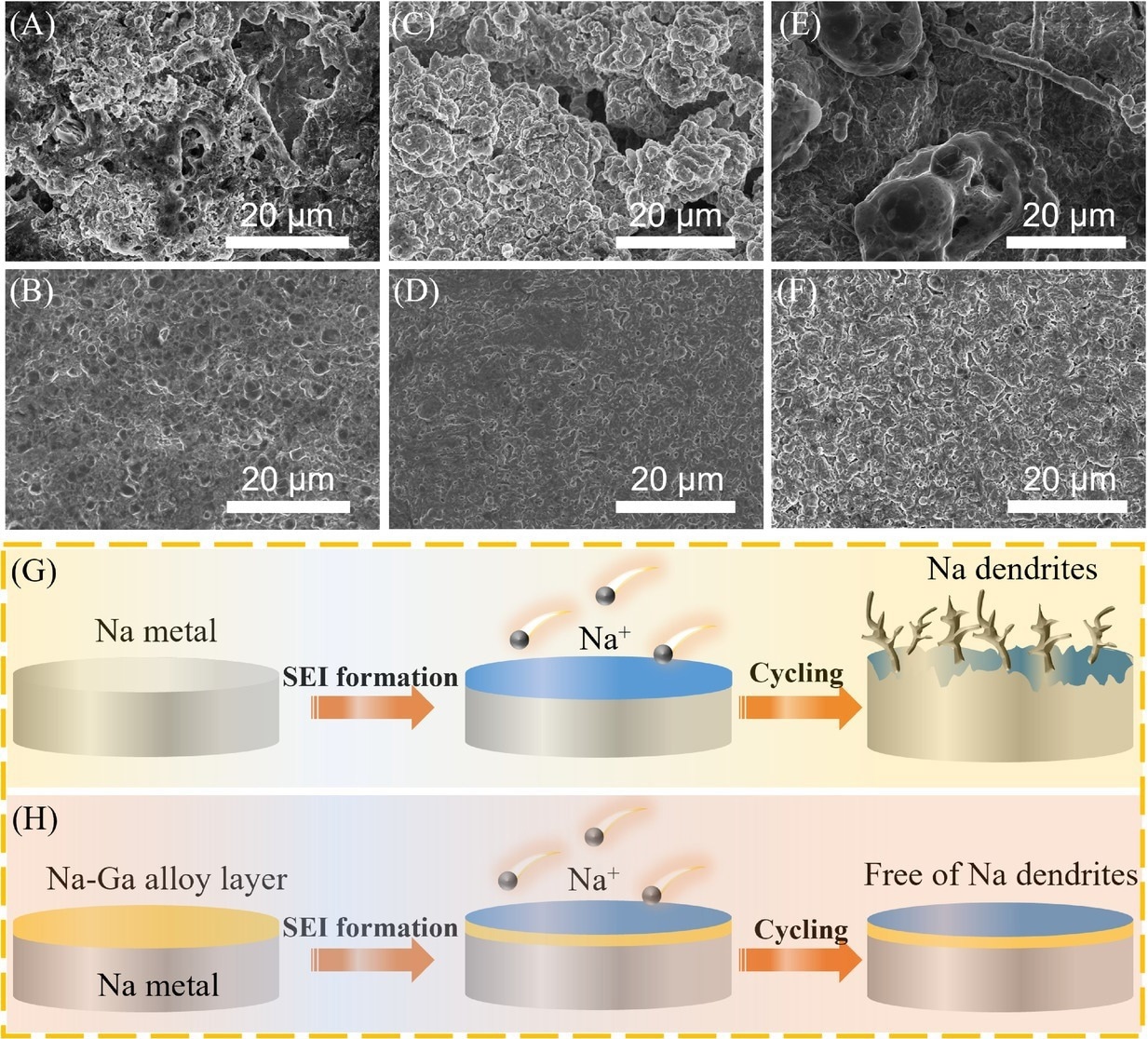
The postmortem scanning electron microscopy (SEM) images analysis for bare Na and Na–Ga alloy layer by rolling the metal Ga on Na metal (NGAL-Na) at 1 mA cm−2 with various plating/stripping capacities: (A and B) 1 mAh cm−2 after 10 cycles, (C and D) 3 mAh cm−2, and (E and F) 6 mAh cm−2 after five cycles. (G and H) Schematic illustrations of Na deposition behaviors of Na and NGAL-Na electrodes. SEI, solid electrolyte interphase. Image Credit: Lv, X et al., SusMat
A dense and flat morphology was observed in NGAL-Na, with a 45 µm-thick sodium-gallium alloy layer. The elements of gallium and sodium were distributed uniformly on the sodium metal surface, indicating the formation of a homogeneous sodium-gallium alloy layer.
The fabricated alloy layer displayed exceptional sodiophilicity, which enhanced long-term stability in the sodium metal anode by effectively inhibiting the sodium dendrite growth and protecting the sodium metal.
Moreover, the use of NGAL-Na electrodes considerably improved the electrochemical performance and lifetime of full cells and symmetric cells. For instance, the symmetric NGAL-Na cell worked on different sodium stripping/plating capacities in carbonate electrolytes, such as at one mA cm−2/ 6 mAh cm−2 plating/stripping capacity for a long lifetime of 468 hours.
Similarly, the NGAL-Na||NVP full cell realized a satisfactory rate performance and cyclic stability, delivering 100 mAh g−1 capacity at 10 C after 500 cycles under ambient temperature, and a lower voltage polarization.
To summarize, the findings of this study demonstrated the feasibility of fabricating a sodium-gallium alloy layer on sodium anodes to stabilize these anodes in sodium metal-based batteries. This strategy could also be used to improve the electrochemical performance and stability of metal electrodes to develop stable and safer metal-based rechargeable batteries.
Reference
Lv, X., Tang, F., Yao, Y. et al. (2022) Sodium–gallium alloy layer for fast and reversible sodium deposition. SusMat. https://onlinelibrary.wiley.com/doi/10.1002/sus2.97
Disclaimer: The views expressed here are those of the author expressed in their private capacity and do not necessarily represent the views of AZoM.com Limited T/A AZoNetwork the owner and operator of this website. This disclaimer forms part of the Terms and conditions of use of this website.