Lithium metal anodes are considered highly promising as anode materials for lithium-based batteries. However, the unavoidable growth of dendrites on the lithium anode leads to issues in advancing the practical uses of lithium metal batteries. It is imperative to gain a precise understanding of the lithium dendrite growth mechanism.
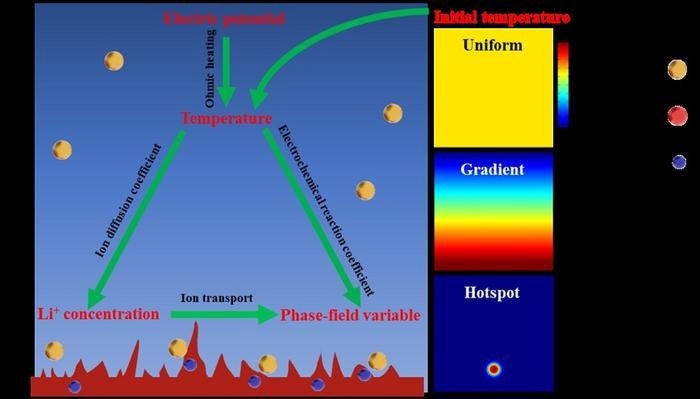
This picture illustrates the effects of temperature on Li electrodeposition, highlighting both its direct influence and indirect influence via Li+ concentration and electric potential on Li dendrite morphology (described by phase-field variable). Image Credit: Siqi Shi, Shanghai University
The study was published on September 12, 2023, in the Energy Material Advances journal.
The great electrochemical phase-field simulation efforts devoted to exploring the dendrite growth mechanism under the temperature field recently. The uniformity of temperature distribution inside batteries has a substantial impact on the stability of Li electrodeposition and dissolution, and the mechanism underlying the temperature-dependent Li dendrite growth remains controversial.
Siqi Shi, Study Author, School of Materials Science and Engineering, Shanghai University
Shi mentioned that a range of simulation methods is employed to study lithium dendrites at various scales, including density functional theory, molecular dynamics, kinetic Monte Carlo, and the phase-field approach.
Siqi Shi says, “Phase field simulation has emerged as a pivotal tool for comprehending temperature-dependent Li dendrite growth dynamics and multiple physical fields, and excels in addressing intricate morphological evolution and coupling of multiple physical fields.”
In our previous work, we have proposed specific strategies to suppress dendritic growth by adjusting separator pore size/thickness/surface coating particles via phase field simulation. Besides, we incorporated the dependent relationship among diffusion coefficient, exchange current density, applied electric potential, electrolyte concentration, and temperature into the electrochemical phase-field model to capture their synergistic effects on electrodeposition morphologies.
Siqi Shi, Study Author, School of Materials Science and Engineering, Shanghai University
“This phase-field simulation is closer to the practical electrodeposition process and provides rational guidance for designing high safety lithium-based batteries,” Shi explained.
“However, this simulation method still has some problems. The reliance of this model on experimental data limits its applicability to other battery systems,” Shi stated.
Simultaneously, the study examined the influence of initial temperature on lithium dendrite morphology by considering temperature-dependent ionic diffusion coefficients, reaction coefficients, and conductivity.
However, it did not incorporate the temperature field. Researchers incorporated the temperature field to explore how the initial temperature affects lithium dendrite morphology, providing a unified explanation for the seemingly conflicting effects of temperature increase on dendrite formation, inhibition, and the dual effects observed in different electrolyte types.
Maybe it is necessary to comprehensively considering the temperature-dependent Li+ diffusion coefficient, electrochemical reaction coefficient, and initial temperature distribution in phase-field model.
Siqi Shi, Study Author, School of Materials Science and Engineering, Shanghai University
In 2018, a heat transfer-based electrochemical phase-field model was initially developed. It incorporated the temperature-dependent Li+ diffusion coefficient and identified that both internal self-heating and an elevated, uniformly distributed initial temperature could suppress dendrite growth.
More recently, a precise thermally-coupled model was developed by incorporating the experimental temperature-dependent conductivities of the electrode and electrolyte, surface tension, reaction rates, and diffusion coefficients. This research revealed that increasing the temperature promotes dendrite formation.
“Diverse strategies to regulate Li dendrite growth have been developed, including modifications to a specific battery component and applications of external fields such as pressure, magnetic field, acoustic wave, light field, electric field, and temperature field. In this paper, we establish a mechanism diagram correlating the activation–energy ratio, uniform initial temperature, and maximum dendrite height, which unifies the seemingly contradictory simulation results,” Shi noted.
“We surmised that, based on nonuniform initial temperature distribution of Lithium dendrite, a positive temperature gradient along the discharging current facilitates uniform Li+ deposition and local hotspot should be avoided,” Shi added.
The researchers observed that in the case of a non-uniform initial temperature, their simulations demonstrated that a positive temperature gradient along the discharge current promotes even Li+ deposition. They also noted that local hotspots should be prevented to achieve this uniform deposition.
Shi adds, “The findings of this study provide valuable insights for future advancements in temperature regulation to control dendrite growth.”
“Lithium metal anodes are regarded as highly promising anode materials for Li-based batteries, but the inevitable dendrite growth on the Li anode results in low Coulombic efficiency and other problems,” Shi says. “To further promote the practical application of lithium metal batteries, we need to understand the growth mechanism of lithium dendrites precisely.”
Shi is affiliated with the Materials Genome Institute at Shanghai University. Zhang is associated with the Clean Combustion Research Center (CCRC) in the Physical Sciences and Engineering Division (PSE) at King Abdullah University of Science and Technology (KAUST). Additional contributors to this research include Yajie Li and Wei Zhao from the School of Materials Science and Engineering at Shanghai University.
The study received support from the National Natural Science Foundation of China (grants 52102280 and U2030206), the Shanghai Municipal Science and Technology Commission (grant no. 19DZ2252600), and the Scientific Research Project of Zhijiang Laboratory (2021PE0AC02).
Journal Reference
Li, Y., et al. (2023). Unified picture on temperature dependence of lithium dendrite growth via phase-field simulation. Energy Material Advances. doi.org/10.34133/energymatadv.0053.