Inverse gas chromatography, also known as IGC, is a gas phase technique that was developed over forty years ago to inspect the bulk and surface properties of fibrous and particulate materials.
Until now, most IGC studies have been performed on home-built equipment that often uses semi-automated or manual experimental techniques. This has led to a range of results that are mostly ambiguous because of variations in sample preparation, methodology, instrument design, and individual operator skill.
An experiment includes a sequence of frontal injections or vapor pulses eluting via a column packed with the sample being analyzed. The retention time of the vapor is determined with a flame ionization detector (FID).
Varying the flow rate, vapor probe molecule, column conditions or temperature reveals a wide range of bulk and surface properties of the sample. Figure 1 shows IGC and analytical gas chromatography.
.jpg)
Figure 1. Analytical Gas chromatography and Inverse gas chromatography (iGC)
Surface Measurement Systems has developed an inverse gas chromatography instrument, which is touted to be world’s first commercial system. IGC SEA can be used to determine physico-chemical properties of solid materials such as diffusion kinetics, acid/base/polar functionality of surfaces, surface energies, surface heterogeneity, solubility parameters, thermodynamic work of cohesion and adhesion and phase transition temperature.
Surface Energy
Surface energies are usually determined by liquid wetting angle methods, but these are very complicated to apply reproducibly on free-flowing powders. In contrast, the IGC SEA instrument can be used to calculate the surface energies of powders as it not only eliminates the need for liquid wetting approach, but also removes the necessity for particle compression. Furthermore, the IGC SEA helps in measuring surface energies as a virtue of humidity.
In order to determine the surface energy of solid materials with the IGC SEA instrument, a sequence of pulsed injections is made via the packed sample column with different probe vapor molecules. Moreover, these measurements are performed at infinite dilution where just a few probe molecules are available for surface interaction.
For this reason, only the highest energy sites on the surface are covered which offers the highest sensitivity of the quantified parameters. In the dispersive component of surface energy (D), probe molecules will be a sequence of alkanes having different carbon chain lengths.
Based on the surface tension of the probe gases in their liquid phase as well as their retention behaviour on the solid sample, it is possible to calculate the surface energy of the solid material.
Figures 2 and 3 show the retention behaviour for a set of alkane probes on microcrystalline cellulose at 300K for 0% RH and 70% RH determined with the IGC SEA instrument. The measured DS values for microcrystalline cellulose are 44.6mJ/m2 at 70% RH and 57.0mJ/m2 at 0% RH.
.jpg)
Figure 2. n-alkaline infection on MCC.
.jpg)
Figure 3. Dispersive surface energy plots for MCC.
Moreover, the reduction in dispersive surface energy with humidity can be attributed to the adsorption of water molecules on the surface. This means that the powder can be anticipated to have varied flowability and mixing properties based on the humidity in the environment.
Due to having infinite dilution conditions, the surface energy acquired from IGC SEA measurements is a very sensitive parameter. The interaction with the highest energy sites on the surface allows variations between similar materials, thus making IGC SEA suitable for the identification of batch-to-batch issues and characterization of polymorphs.
Acid-Base Parameters
Besides the dispersive contribution, specific polar interactions must be taken into account because the acid-base chemistry of the surface plays a key role in formulation stages. Alkanes are injected to measure the surface energy’s dispersive contribution.
If polar probe molecules are also injected, it is possible to achieve acid-base parameters. In the case of the polar probe molecules, the experimental points will be placed further than the alkane straight line in the surface energy plot.
The vertical distance between the straight line and each point (Figure 4) denotes the specific contribution of the interaction that is expressed in the specific free energy, ΔG.
.png)
Figure 4. Determination of specific free energy of polar probes
By utilizing established concepts such as van Oss or Guttman, it is possible to determine acid-base parameters, as shown in Figure 5. The acid-base parameters can be used to differentiate the surface chemistry of an excipient or drug and can even be utilized to predict drug-carrier interactions.
.jpg)
Figure 5. Dispersive surface energy plots for MCC.
Matrix Interactions
In the present example, three thermoplastic polymers and three different carbon fibres (untreated, oxidized, and sized and oxidized) were inspected using the IGC SEA instrument. Figure 6 shows the specific and dispersive surface energies achieved.
.jpg)
Figure 6. Dispersive, specific and total surface energies for carbon fibres and polymers.
Experiments were performed at infinite dilution, i.e., at low concentrations of vapor interacting with high energy sites on the surface, which elucidates the high total surface energies. The infinite dilution regime, however, is highly sensitive to even slight changes and hence effectively applied for examining batch-to-batch issues.
The surface energy of the components can now be utilized to assess the interaction between each pair of polymer and fibre. Such an estimation is achieved by measuring the work of adhesion, WA by applying the following equation:
WAtotal = 2(1d * 2d)1/2 + 2(1sp * 2sp)1/2
Numbers 1 and 2 represent polymer and fibre, respectively. To draw a comparison, adhesion measurements have been performed using the fibre pull out test. The results obtained from these measurements have been correlated with the values for the work of adhesion projected from surface energy measurements.
The determined acid-base parameters of the untreated and treated carbon fibres clearly represent the effect of the oxidation on the carbon fibre surface. The surface acidity increased due to an increase in electron capacity of the oxidized fibre, as shown in Figure 7.
.png)
Figure 7. The acid-base parameters of carbon fibres as order to represent the surface acidity/basicity.
Please click here if you would like more information on the instrument used in this article or a quote
Glass Transition Temperature
IGC SEA is a highly sensitive technique that can be used for detecting and determining first and second order phase transitions like melting and glass transitions, respectively. Originally, at low temperatures the interaction between polymer and vapour probe is controlled by surface adsorption (A-B).
Upon increasing the temperature, more and more bulk absorption takes place because of increased molecular motion. The latter is a kinetically controlled process and a deviation can be observed from linearity (B-C) (Figure 8).
.png)
Figure 8. Schematic illustration of glass and melting transition in an IGC SEA retention diagram.
The temperature of glass transition depends on material properties and environmental conditions such as morphology and humidity.
Contrary to the traditional techniques used in the characterization of Tg, such as dynamic mechanical thermal analysis (DMTA) and differential scanning calorimetry (DSC), IGC SEA offers a precise and sensitive technique to study Tg under diverse relative humidity conditions.
In the present example, the glass transition temperature of PVP K25 was established at 45% RH. Figure 9 shows the retention curve in the vicinity of the glass transition.
.png)
Figure 9. Glass transition of PVP K25 at 45% RH, measured with 1-butanol as vapour probe
Hildebrandt Solubility Parameters
The solubility parameter, also known as cohesion parameter, was first defined by Scatchard and Hildebrand to explain the interactions between polymers and solvents using thermodynamic theory of standard solutions.
A wide range of techniques are available to determine the solubility parameters, which are either based on the Hildebrand theory or the Hansen theory to measure the total solubility parameter.
.png)
Figure 10. Solubility parameter plot for PMMA at 363.15 K. The different experimental points represent the various probe molecules used.
In the present example (Figure 10), the Hildebrand solubility parameter has been established for polymethyl methacrylate (PMMA). In this analysis, retention volumes are calculated for a range of vapor phase probe molecules having a significant bulk interaction with the solid component.
Solubility parameters can easily predict the absorption of complex drug molecules or simple solvents across a wide range of substrates. They have also been correlated with nanoparticle formation and oral absorption.
Surface Energy Heterogeneity
Real solids have a range of higher and lower energy sites on their surfaces owing to the presence of various surface functional groups, surface irregularities, and surface topographies.
In this analysis, complete surface energetic of D-mannitol - a model pharmaceutical excipient - with and without surface methylation was measured with the IGC SEA instrument (Figures 11 and 12).
.jpg)
Figure 11. Dispersive surface energy profiles (as a function of surface coverage).
.jpg)
Figure 12. Specific (acid-base) surface energy profiles
D-mannitol is an excellent example as it has a high degree of inhomogeneous surface on the native mannitol crystal. After the metylation, the surface was completely covered with dimethyl-silane groups (making the surface homogenous/uniform).
SEA surface energy profile measurements are able to distinguish the degree of homogeneity (or heterogeneity) and changes in the surface chemical environment. Figure 12 highlights how the silanized D-mannitol became an isotropic hydrophobic surface property.
Conclusion
The IGC-SEA instrument from Surface Measurement Systems is an advanced inverse gas chromatography technique that has been specifically developed to measure the heterogeneity of surface energy.
The system can also be used to determine bulk and surface properties of solid materials such as heat of sorption, glass transition temperature, adsorption isotherms, and solubility parameters. In addition, surface energy and wettability can also be correlated with adhesion properties.
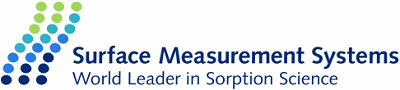
This information has been sourced, reviewed and adapted from materials provided by Surface Measurement Systems Ltd.
For more information on this source, please visit Surface Measurement Systems Ltd.