Sponsored by AdmesyJun 24 2015
Raman spectroscopy can be described as a molecular type of spectroscopy which is capable of detecting and differentiating material’s unique fingerprint. Typical Raman applications are in chemistry.
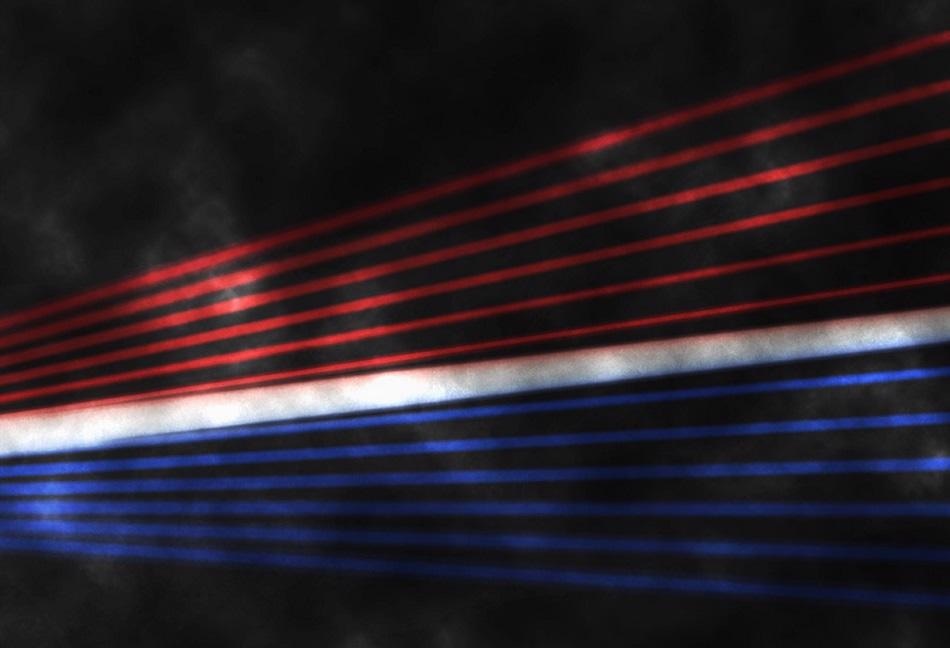
Essentially, a Raman spectrometer consists of a monochromatic light source, for example, a laser and spectrometer. The narrow banded light source is projected towards a sample which is measured by means of the spectrometer.
From that perspective, the measurement principle can be considered a reflective measurement. This is in contrast to other material determination methods based on absorption.
The Raman Effect
The high energy level of the light source causes the so-called spontaneous Raman effect: a form of inelastic light scattering caused by the incident electromagnetic waves of the light source, which changes the vibrational energy levels of the molecule.
Due to these changes in vibrational energy levels, a part of the incident light is shifted towards other wavelengths (or frequencies). These shifts are called inelastic scatterings and can be a decreased or increased shift, known as Stokes or Anti-Stokes shift respectively.
Next to the inelastic scatterings, elastic scattering can occur. The latter are scatterings of exactly the same wavelength of the narrow banded light source, and are known as Rayleigh scattering. The graph below shows an example of the three types of scattering, both elastic and inelastic in perspective of vibrational energy levels.
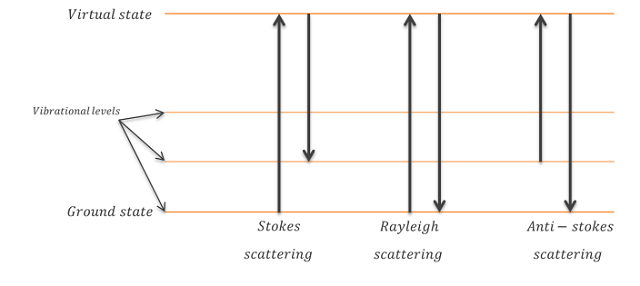
Image Credit: Admesy
The intensity of the shifted wavelengths, and the amount of shifting, is dependent on the molecule. Each molecule has its own, unique shift in wavelength and intensity of shifted wavelengths — a kind of fingerprint.
These fingerprints can be plotted as graphs, and data can be logged in libraries for future use. That way, materials can be identified by comparing the measured fingerprint with fingerprints stored in the library, revealing directly what type of molecules are detected.
Collecting Data
As straightforward and easy it may sound, gathering usable data by means of Raman spectroscopy is quite challenging. One of the major difficulties is gathering the inelastic scattering which provides the typical fingerprint. The sample which is measured does not only reflect both types of scattering, but also in a rather undesired proportion: The majority of the scattering is Rayleigh scattering. Only a very small amount, approximately 10-5 % of the light, is inelastic scattering.
In that perspective, the inelastic scattering signal is very weak, making it hard to distinguish molecules. Weak signals can typically be compensated for with longer integration times. A very well-designed dark compensated spectrometer is essential for such applications. Due to the large amount of Rayleigh scattering, however, the signal will directly saturate and become unusable to identify molecules when using long integration times.
A short integration time will lead to poor a signal-noise ratio, which is inappropriate to carry out accurate measurements. Wavelength shifts can hardly be distinguished from noise. Obviously, the wavelength range of the light source is excluded from the measurement results. Proper elimination of the Rayleigh scattering, therefore, is essential for Raman spectroscopy.
This also explains the reason for using narrow banded light sources. A monochromatic light source only takes away a very small part of the wavelength range, thus leaving most of the wavelength range for measurement and possibility to detect shifted scattering. Typically, the graphs showing a molecules fingerprint are plotted from the Rayleigh scattering at 0 point. The Rayleigh band is at 0-1 cm.
Realizing that a monochromatic (typically laser) light is most appropriate for Raman spectroscopy, the choice for a particular wavelength depends largely on the application. A shorter wavelength will lead to a more powerful Raman signal, and would therefore be considered an advantage. Most organic molecules, however, will show fluorescent effects when lit by short wavelengths.
These shifts may interfere with the inelastic scattering, and may lead to wrong interpretation of measured samples. Thus, lasers with different wavelengths can lead to entirely different Raman spectrums.
Conclusion
For chemical and other applications that require molecule identification, Raman spectroscopy offers a number of advantages. First of all, only a very small volume of a material is needed for proper measurements. Areas less than 1 µm in diameter are sufficient for molecule detection. Additionally, samples can be measured directly. There is no need for sample preparation, thus saving time.
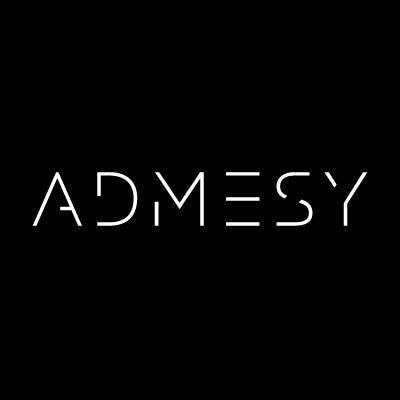
This information has been sourced, reviewed and adapted from materials provided by Admesy.
For more information on this source, please visit Admesy.