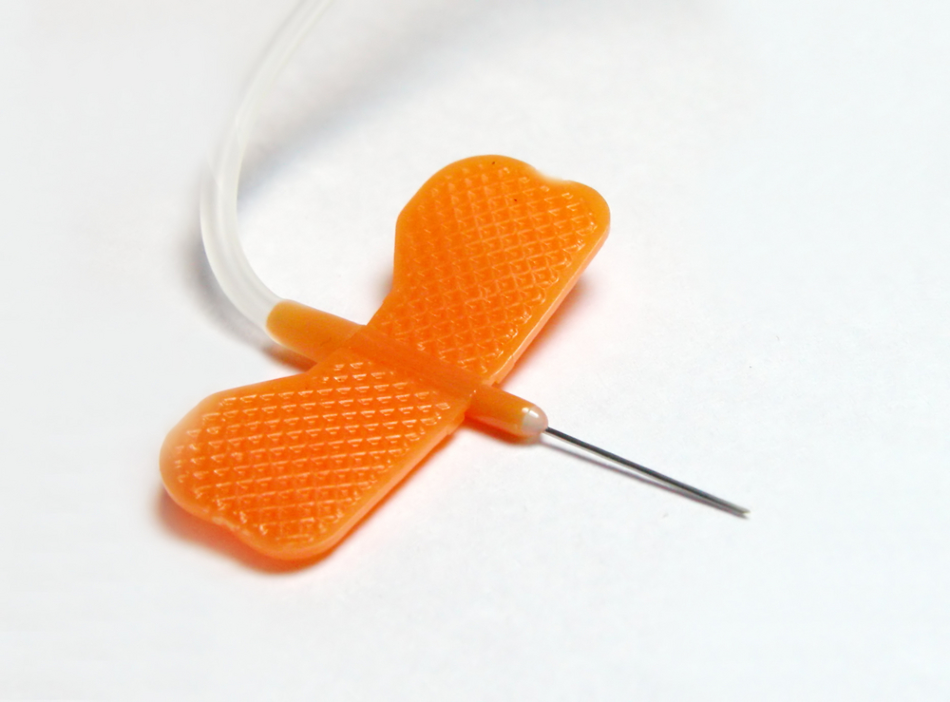
Image Credit: Radu Razvan/Shutterstock.com
Hyperbranched polymers (HBPs) are a class of dendritic polymers that have been incorporated into many different electronic and biomedical applications. More recently, the unique features of HBPs have gained a considerable amount of attention for their potential use as drug delivery systems.
What are Dendritic Polymers?
Dendritic polymers are highly branched molecules that consist of three-dimensional architectures closely resembling that of a tree. The six different classes of dendritic polymers include dendrimers, hyperbranched polymers (HBPs), multi-arm star polymers, dendronized or dendrigraft polymers, hypergrafted polymers and dendritic-linear block polymers.
The unique architecture of dendritic polymers includes a larger number of terminal functional groups as compared to linear polymers. Dendritic polymers are also associated with several advantageous chemical properties, some of which include good solubility, impressive biodegradability and biocompatibility, versatile functionalization, high density of terminal functional groups, nanometric size and low melt viscosity. Overall, these characteristics have allowed dendritic polymers to be used for various applications within biological and biomedical fields. Some of the recent successful bioapplications of dendritic polymers can be found in drug delivery systems, gene transfection, protein delivery, bioimaging, biomineralization and tissue engineering.
Advantages of HBPs
Although a considerable amount of research has been conducted on the dendrimer class of dendritic polymers, these highly branched symmetrical macromolecules are also associated with lengthy, tedious and expensive preparation requirements, thereby limiting its versatility for scientific investigation.
Comparatively, HBPs are highly and randomly branched macromolecules that can be easily prepared at a much more inexpensive cost to the user. In addition to being cost-effective, HBPs are associated with many other advantageous properties, some of which include a low tendency to chain entanglements, smaller hydrodynamic radius and a high degree of branching. These qualities of HBPs have allowed HBPs to be used for a wide range of applications including biomedicine, electronic, optical and magnetic materials, coatings, additives and supramolecular chemistry.
HBPs in Drug Development
Some of the latest trends in drug discovery and development have involved the effective use of nanocarriers as drug delivery systems. Nanocarriers, which are typically in the size range of 1 to 100 nanometers (nm) in diameter, are associated with ideal biocompatibility properties and enhanced affinity for target sites. Several different nanocarriers have been evaluated for their efficiency in transporting both hydrophilic and hydrophobic drugs, some of which include inorganic nanocarriers, such as carbon nanotubes and quantum dots, as well as organic lipid nanocarriers, such as liposomes, dendrimers and HBPs.
The branching, linear and terminal units of HBPs are highly flexible, thereby allowing researchers to manipulate the structure and/or responsiveness of these units during the design of nanocarriers for drug delivery. These units can be altered to enable responses to a single stimulus or multiple stimuli, of which can include, but are not limited to, temperature, redox, light, enzymes or pH. Additionally, the globular 3D structure of HBPs is ideal for encapsulation of small-molecule drugs, such as those used in cancer treatment, or radioisotopes for diagnostic imaging purposes.
Drug Loading using HBPs
The encapsulation of drugs by HBPs has been achieved through several different methods. Several different types of non-covalent interactions, such as hydrophobic interactions, hydrogen bonding, ionic interactions and steric trapping within a crosslinked network, have been used to load drugs into polymer-based drug delivery systems like HBPs. This type of drug loading technique can enhance the interactions between the drug and the HBP to improve the drug’s stability, provide high drug loading capabilities and manipulate the drug delivery profile of the encapsulated molecule as needed.
Passive Targeting of HBPs
Passive targeting is another type of drug delivery design that has allowed HBPs to optimize the pharmacokinetics of a drug to ultimately promote their transport to target tissues. This improvement in drug targeting is achieved by increased circulation of the drug within the bloodstream, thereby allowing more highly vascularized tissues, such as tumors, to become easily accessible to drug-loaded HBP nanocarriers.
Notably, unimolecular HBPs are associated with sizes that are too small for passive drug targeting, and as a result of this, a considerable amount of work has been done to investigate the potential of HBPs as nanocarriers for bioimaging molecules. The small size of unimolecular HBPs, while a limiting factor for chemotherapeutic options, has been shown to reduce the toxicity of isotopes traditionally used for diagnostic imaging by enhancing their elimination through urine and feces.
Active Targeting of HBPs
Despite the advantages associated with passive targeting, it also experiences certain limitations, such as a low interaction with the cell surface that results in inefficient diffusion of the nanocarrier into tumor cells. Comparatively, active targeting of HBPs has demonstrated an ability to improve the efficient delivery of encapsulated drugs while simultaneously enhancing cell specificity.
Active targeting often involves the incorporation of targeting moieties directly onto the surface of the nanocarriers. These moieties allow for the receptors present on cancer or angiogenic endothelial cells to immediately recognize targeted nanocarriers, thereby resulting in the binding of these molecules to target cells of interest and ultimately internalization of the drug delivery system. Some of the most recent targeting moieties that have been conjugated on HBPs for therapeutic purposes include peptide and oligonucleotide aptamers and folic acid.
References and Further Reading
Ma, Y., Mou, Q., Wang, D., Zhu, X., & Yan, D. (2016). Dendritic Polymers for Theranostics. Theranostics 6(7); 930-947. DOI: 10.7150/thno.14855.
Paleos, C. M., Tsiourvas, D., Sideratou, Z., Tziveleka, L. A. (2010). Drug delivery using multifunctional dendrimers and hyperbranched polymers. Expert Opinion in Drug Delivery 7(12); 1387-1398. DOI: 10.1517/17425247.2010.534981.
Chamundeeswari, M., Jeslin, J., & Verma, M. L. (2018). Nanocarriers for drug delivery applications. Environmental Chemistry Letters 17; 849-865. DOI: 10.1007/s10311-018-00841-1.
Kavand, A., Anton, N., Vandamme, T., Serra, C. A., & Chan-Seng, D. (2020). Synthesis and functionalization of hyperbranched polymers for targeted drug delivery. Journal of Controlled Release 321; 285-311. DOI: 10.1016/j.jconrel.2020.02.019.
Disclaimer: The views expressed here are those of the author expressed in their private capacity and do not necessarily represent the views of AZoM.com Limited T/A AZoNetwork the owner and operator of this website. This disclaimer forms part of the Terms and conditions of use of this website.