AFM has been a vital and exceptionally informative technology for investigating biomaterials and chemical processes at nanometre dimensions ever since its development in 1986. This article will go through the fundamentals of AFM and how it is used to analyze biopolymers.
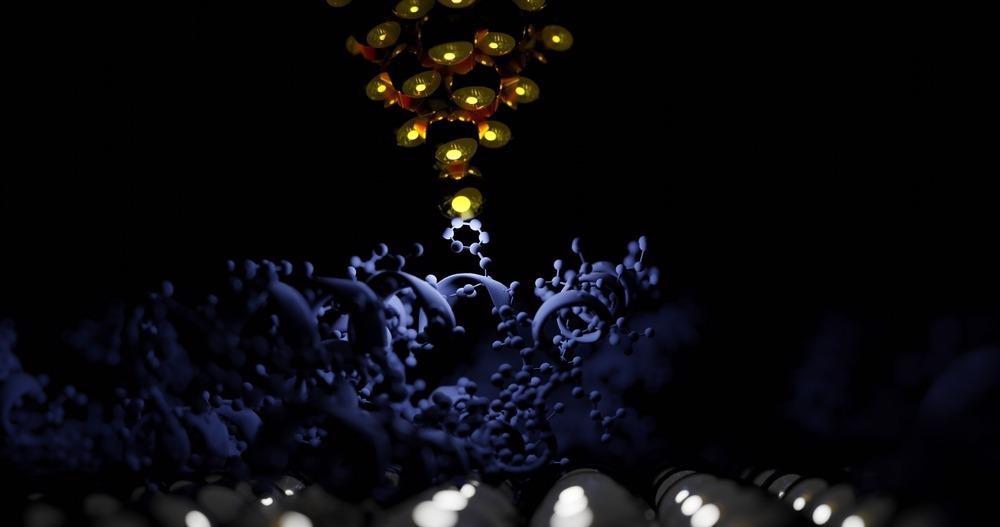
Image Credit: sanjaya viraj bandara/Shutterstock.com
Introduction to Biopolymers
Biopolymers are polysaccharides that come from natural sources and are not the same as manufactured biodegradable materials. Some biopolymers have been discovered to have ionic and electronic conductance and are as such referred to as electro-active biopolymers (EABP).
Biopolymers are created using a variety of technologies and procedures which may combine cultivation, filtering, hydrolysis, isomerization, poly-condensation, and dehydration. These compounds have desired qualities such as recyclability, availability, biocompatibility, as well as other special properties such as strong adsorption capacities and ease of synthesis.
Applications of Biopolymers and Utilization of AFM
Biopolymers are employed in a wide range of commercial processes, including packaged foods, pharmaceuticals, and healthcare. They can be used in lieu of conventional petroleum-based plastic products in a range of applications. Some biopolymers have also been used in applications where traditional plastics would be ineffective, such as the production of synthetic tissue.
In comparison to synthetic polymers, biopolymers frequently display inferior tensile characteristics, chemical stability, and ease of processing. They can be strengthened with additives to increase their qualities and make them more appropriate for certain purposes. Biopolymer hybrids are biopolymer composites that have been enhanced in this particular fashion. To carefully choose appropriate fillers, AFM might be used in conjunction with other techniques to verify their compatibility.
Preference of AFM over Other Methods
AFM, being among the few technologies with nanoscale precision, enables microscopic viewing of polymeric materials. The key benefit of AFM over light microscopy analysis is its precision and level of detail, where AFM of operational biological molecules in an aqueous medium has been demonstrated to accomplish a resolution of around 1 nm, which is exactly equivalent to the shortest tip radius dimension.
AFM pictures may be captured in the ambient atmosphere or liquids at a lower resolution, making it useful for biological tissue—typically water-containing—in contrast to scanning electron microscopy (SEM) which requires a vacuum in the specimen compartments. AFM does not require the dyeing or encapsulation of materials. As a result, preparing the specimen is simple. Additionally, it is also non-destructive in many cases.
Sample Preparation for AMF Application
Depending upon the type of data needed and the specimen being analyzed, the AFM process could include different modes and techniques. Considering the modes of operation, the main ones include tapping mode, contact mode, and non-contact mode. The techniques include phase imaging, attractive and repulsive interaction regimes, nanoindentation, peak force quantitative nanomechanical mapping, Kelvin Probe microscopy, and conductive AFM.
The latest research by Homburg and Ehrmann published in the journal Polymers reveals that the methods described above may be utilized in a wide range of biopolymers and hydrogels deployed in a range of industries for different purposes. Chitosan, cellulose derivatives, carrageenan, alginate, polyesters, enzymes, and DNA are polysaccharides, polynucleotides, and polypeptides, and are frequently used in biotechnological processes.
More from AZoM: Newly Discovered Biopolymer Made From Bacterial Enzyme
To avoid sample degradation, AFM studies on incredibly fragile materials are often done in the tapping or non-contact mode. Aerosol smearing an aqueous solution over a newly sliced mica platform and allowing it to cure by air is a common approach for receiving a smooth surface of a biopolymer.
This approach has been proven for AFM scans of several natural polymers and nanoscale complexes, and it revealed straight and nonlinear triple helices of the polysaccharide’s biopolymer composites. A potential issue with this preparative process is the creation of artifacts such as agglomeration and salt crystallization if the specimen is not thoroughly cleaned after drying.
Atomic Force Microscopy Results of Different Biopolymers
According to Homburg and Ehrmann, AFM is most often utilized for the analysis of chitosan. AFM was used to explore laser-induced cyclic microstructures in chitosan, starch, and chitosan/PVP blends. It was discovered that certain periodical exterior patterns may be formed in amorphous chitosan.
Aside from these topography-related observations, the phase mapping AFM technique on chitosan has been given more attention than on carrageenan. Similar to carrageenan, certain PeakForce QNM data for chitosan has been demonstrated, showing that AFM has been used accurately for its investigation.
AFM on alginate is generally used for anatomical research. Singh et al. employed AFM to assess the morphology of an alginate/sterculia gel that they proposed as a substitute for brain medication delivery. During pH-triggered expanding and deswelling, alginate/chitosan/alginate-modified silica nanoemulsions and chitin layered coatings were studied, and topography evaluations with AFM were employed to confirm that their structural rigidity in PBS was preserved.
In addition, AFM has also been used to explore biopolymer hydrogels. Aside from characterization features, there are also physical characteristics of chitosan hydrogels investigated utilizing forced-distance graphs to estimate the deformation and Peak-Force QNM experiments. The coexistence of two phases in chitosan/carboxymethylcellulose hydrogels was investigated using phase pictures.
In short, atomic force microscopy is vital to the study of biopolymers. In the future, the scope of biopolymers is expected to skyrocket, with AFM playing a crucial role in their analysis.
References and Further Reading
Joshi, J.; Homburg, S.V.; Ehrmann, A. 2022. Atomic Force Microscopy (AFM) on Biopolymers and Hydrogels for Biotechnological Applications—Possibilities and Limits. Polymers. 14, 1267. Available at: https://www.mdpi.com/2073-4360/14/6/1267
Luna, R.; Touhami, F.; Uddin, M.J.; Touhami, A. 2022. Effect of temperature and pH on nanostructural and nanomechanical properties of chitosan films. Surf. Interfaces. 29. 101706. Available at: https://www.sciencedirect.com/science/article/pii/S246802302100777X?via%3Dihub
Smirnov, M. A., et al. 2021. Combined Use of Atomic Force Microscopy and Molecular Dynamics in the Study of Biopolymer Systems. Polymer Science, Series C 63(2). 256-271. Available at: https://link.springer.com/article/10.1134/S1811238221020089
Dubrovin, E. V., and D. V. Klinov. 2021. Atomic Force Microscopy of Biopolymers on Graphite Surfaces." Polymer Science, Series A 63(6). 601-622. Available at: https://link.springer.com/article/10.1134/S0965545X2106002X
Singh, Baljit, and Ajay Kumar. 2020. Synthesis and characterization of alginate and sterculia gum based hydrogel for brain drug delivery applications. International Journal of Biological Macromolecules 148. 248-257. Available at: https://www.sciencedirect.com/science/article/pii/S0141813019396849
Disclaimer: The views expressed here are those of the author expressed in their private capacity and do not necessarily represent the views of AZoM.com Limited T/A AZoNetwork the owner and operator of this website. This disclaimer forms part of the Terms and conditions of use of this website.