The primary role of a display is to look good. This is true for most use cases, and it is essential to ensure that the observer perceives the display as intended. Assessing this perception requires measurement of the display.
This measurement and assessment can take different forms. One can look at the maximum luminance or one can assess the contrast function or tone gradation – the latter often described as a gamma value for displays. This value tends to impact perceived brightness more than differences in absolute luminance. This is because a user adapts to the overall brightness level.
The logical approach to assessing image quality would likely involve measuring a complete image, but for it to be accurate, it would be necessary to separate out the influence of the image data and the impact of other factors such as ambient lighting effects on the measurement.
Taking these factors into account can be very complex, so rather than trying to assess the image quality as a whole, many applications opt to use simplified test images to evaluate single aspects of image quality, such as color, uniformity, contrast, accuracy and gamut.
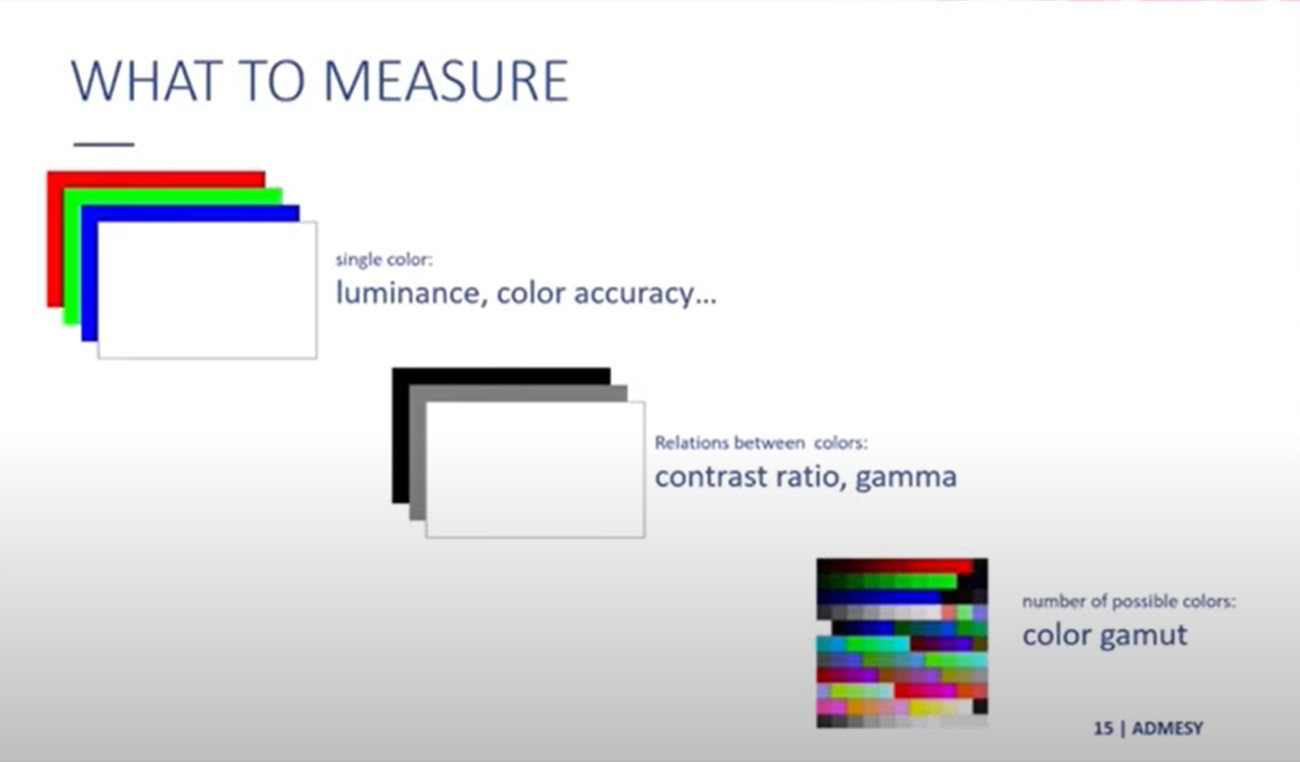
Image Credit: Admesy
Understanding Color Perception
Humans’ color perception depends on many parameters. Especially relevant are the viewing field, surroundings and the luminance level. But also the health and age of the observer contributes to the color perception of an individual observer.
As so many parameters can affect the perception of color, tests should use standardized viewing conditions such as a 2° and 10° viewing field, surroundings and absolute luminance. These standard factors should be in line with the characteristics of the average observer.
Color distribution on the retina is not equidistant nor random. Different parts of the retina are responsible for the short wavelength, middle and long-wavelength light, which makes up different colors. As these differ in terms of their distribution on the retina, it is necessary to use a 2° observer to describe a small viewing field and a 10° observer to describe a larger viewing field. For most applications, for example when an image is viewed on a display, the 2° observer is the better fit.
The sensitivity of these different areas of the retina can be understood mathematically using the so called 'color matching functions'. From those and the light stimulus finally, the CIEXYZ is derived. These values describe the stimulus that appears and the sensation in the eye itself.
One advantage of the CIEXYZ approach is that this is an additive measure, functioning similarly to CRTs and, to a lesser degree, more contemporary displays.
It is important to note that XYZ describes the stimulus in the eye, but not the visual perception. The stimulus is transferred to the brain, where it is processed and where colors are perceived as either bright or dark and as red or green, yellow or blue, or something in between. For example, a grey color will have no or only little yellowish or bluish tint.
These types of perceptions tend to be exclusive. For example, a color perceived as bright cannot also be perceived as dark, or a color perceived as red cannot be perceived as green at the same time. This phenomenon is Hering’s opponent color theory.
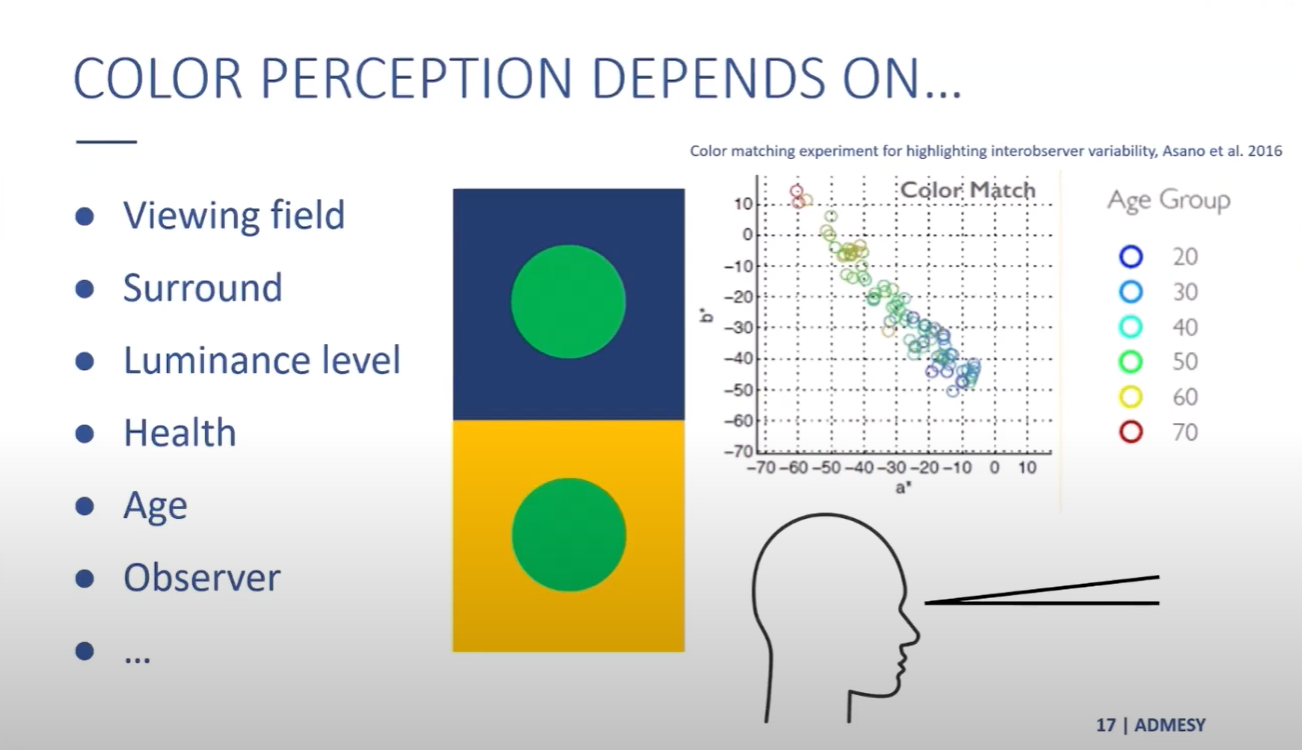
Image Credit: Admesy
Characterizing Color Perception
The CIELAB color space - also referred to as L*a*b* - is a means of describing the human perception of color. CIELAB is calculated relative to a reference white. Using this model, lightness perception (L*) is measured from 0 to 100, while chromatic differences in the a* and b* axis are represented as negative and positive up to around 150.
CIELAB correlates well with human perception of color, and it is possible to use this model to define or find a color difference inside a color space.
When two colors are specified in the CIELAB color space the difference between these colors can simply be calculated via the Euclidean distance – a three-dimensional form of Pythagoras.
This color difference is called 'Delta E'. The Delta E value represents the difference between two colors. The word 'Delta' stands for distance and 'E' comes from the German word 'Empfindung', which translates to 'perception'.
For example, comparing very different colors like blue and green would yield higher Delta E values than comparing two different shades of blue.
CIELAB is roughly perceptually uniform and equidistant, but it is not perfect. There was a need for a more reliable formula that uses weighting factors to take into account different parts of the hue and correct for color perception in different hue regions.
The result of this need for an advanced formula is Delta E 2000, which offers an improved match to human color perception.
It is advisable to always use Delta E 2000 when looking to acquire a color difference. The simple Delta E calculations should nowadays only be employed when looking to comply with an old standard.
There is no linear conversion factor between Delta E and Delta E 2000. But both are close for near-neutral colors, because then the hue and saturation-dependent correction factors which Delta E 2000 uses have little effect.. Delta E 2000 and CIELAB are well suited to evaluating displays, especially when checking for uniformity, color accuracy, and differences in tonal reproduction but also to describe the color gamut — the range of colors a display can reproduce.
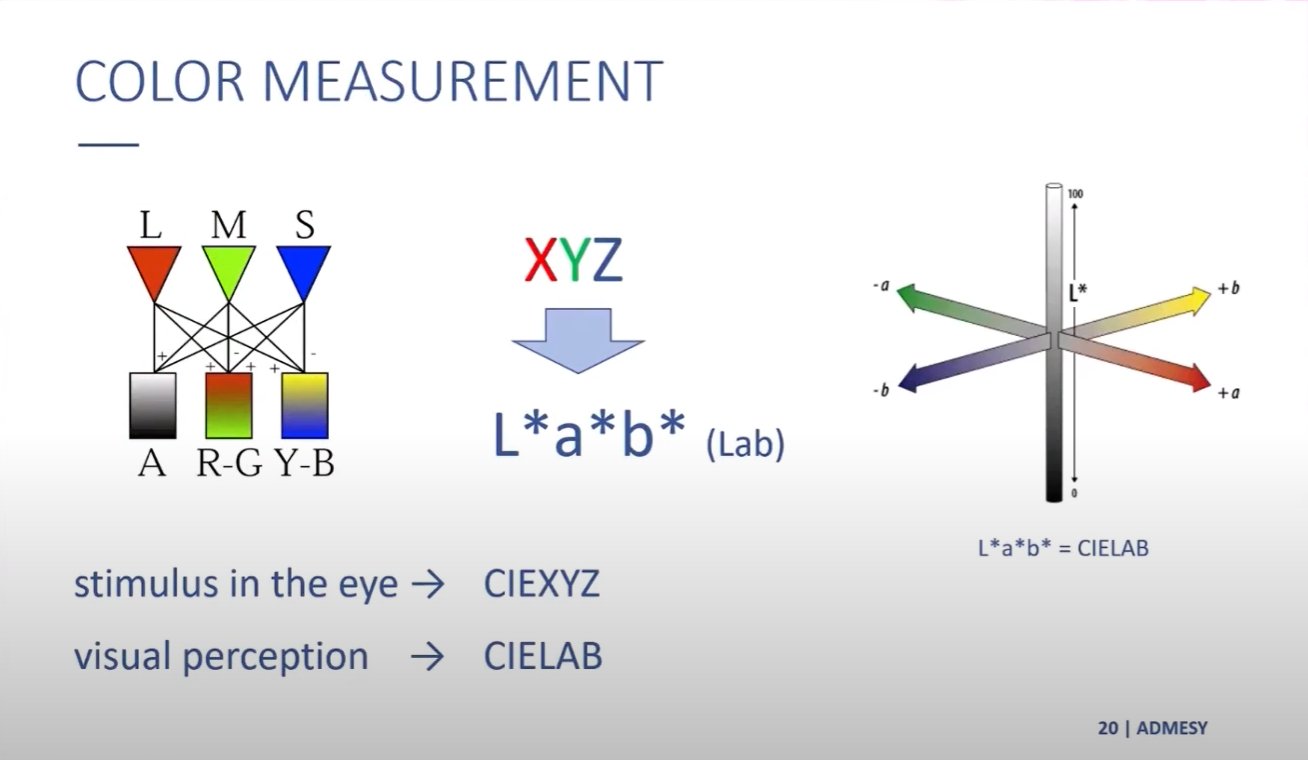
Image Credit: Admesy
Choosing the Best Metric
XYZ or the derived xy chromatic values, like those used in the chromaticity diagram, are useful metrics when characterizing different primary colors, when additivity is important, or where it is necessary to describe the absolute luminance (Y value).
CIELAB is a better option when considering human color perception when determining color differences and in applications where the three-dimensional aspect of color is important. CIELAB is also ideal when comparing normalized lightness.
When characterizing color differences, it is important to use a laboratory-grade instrument. Using simpler instruments will likely lead to clearly visible variations from the instrument itself, making it difficult to judge color differences.
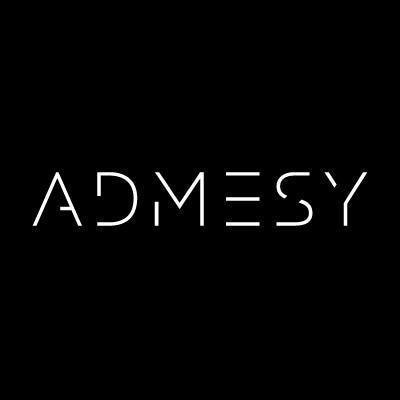
This information has been sourced, reviewed and adapted from materials provided by Admesy.
For more information on this source, please visit Admesy.