For children throughout the world, commercialized baby foods sometimes become their primary source of nutrition and energy. Hence, their quality and safety are critical during these important phases of growth. Toxic elements include arsenic (As), cadmium (Cd), mercury (Hg), and lead (Pb).
They have been labeled toxic to human health by the U.S. Food and Drug Administration (U.S. FDA) and the World Health Organization (WHO), especially for newborns and children who are developing neurologically.
Ingredient crops, including rice, fruits and vegetables, or animals can absorb these elements from the air, water, or soil during growth, and/or introduce them as pollutants during processing and storage.
The “Baby Food Safety Act of 2021” was presented in the United States House of Representatives in March 2021 to limit the inclusion of harmful components in newborn and toddler food items.
Inorganic arsenic (10 ppb, 15 ppb for cereal), lead (5 ppb, 10 ppb for cereal), cadmium (5 ppb, 10 ppb for cereal), and mercury (2 ppb)1 are the hazardous metals with the highest maximum amounts.
Following this, the U.S. Food and Drug Administration (FDA) issued a “Closer to Zero” action plan, which lays out the steps the agency should take over the next three years (and beyond) to decrease hazardous components in foods consumed by infants and young children to as low a level as feasible.
The FDA has issued Elemental Examination Manual (EAM) 4.7, an elemental analytical technique by ICP-MS that includes the analysis of the aforementioned hazardous elements as well as adding trace elements frequently classified as nutrients, such as selenium (Se), zinc (Zn), copper (Cu), and manganese (Mn).
ICP-MS is a strong elemental analysis technology that has multi-element detection capabilities, low detection limits, fast analysis speed, and a large linear dynamic range, among other advantages. Interferences in the form of plasma, matrix-based polyatomic, and doubly-charged ion interferences, as with every analytical method, impact it.
Polyatomic interferences are produced by the interaction of matrix ions with plasma-based ions such as Ar, C, N, O, and H, which can overlap target ions, with examples being 40Ar 35Cl+ on 75As+, 40Ar 12C+ on 52Cr+, 40Ar15 N+ on 55Mn+, 40Ar 38Ar+ on 78Se+, and so on.
Secondary ionization of substances with low second ionization potentials, like rare earth elements, produces doubly-charged ions (REEs). The doubly-charged ions from 150Nd++ and 150Sm++, for example, may interfere with 75As+, while the doubly-charged ions from 156Gd++ and 156Dy++ could interfere with 78Se+.
Since food ingredients (including infant foods) can come from a wide range of geological locations as a result of globalization, the presence of rare earth elements in some foods should not be overlooked for correct elemental analysis.
The Universal Cell Technology (UCT) of PerkinElmer’s NexION® ICP-MS series allows it to operate in Collision mode with kinetic energy discrimination (KED) and Reaction mode with dynamic bandpass tuning (DBT).
A chemically inert gas, typically helium, clashes with both the analytes and interferences in Collision mode. Polyatomic interferences have a bigger cross-sectional diameter than elemental ions of the same mass (e.g., 40Ar35Cl+ is larger than 75Cl+), therefore they collide more and lose more energy, and so cannot get through the energy barrier at the collision/ reaction cell’s exit.
Collision mode, on the other hand, cannot resolve doubly-charged ions. To adjust for interferences from doubly-charged ions, a few strategies have been proposed, which either entail lengthy post-analysis mathematical corrections or sacrifice sensitivity using half-mass corrections.
In this study, As and Se were analyzed using the reaction mode with oxygen as the reaction gas, in which As and Se react with oxygen to create 75As 16O+ at mass 91 and 78Se 16O+ at mass 94, respectively and move away from the respective doubly-charged interferents by +16 amu.
Apart from resolving the doubly charged ion interferents, Reaction mode also provides the lowest detection limits for As and Se without affecting their sensitivity. In some circumstances, Reaction mode can also be utilized to deal with polyatomic interferences, resulting in enhanced sensitivities and detection limits.
Reaction mode with ammonia gas, for example, can be used to eliminate polyatomic interferences on 52Cr, in which the interferents 40Ar 12C+, 35Cl 16OH+, 35Cl 17O+, 37Cl 14N1 H+, and others react with NH3 through a charge-transfer reaction, losing their charge to NH3, and then being rejected in the analyzer quadrupole.
The NexION ICP-MS series was used to analyze Cr and Mn using Reaction mode with ammonia gas and Collision mode with helium to illustrate the adaptability of the NexION ICP-MS series in handling spectral interferences.
This article outlines a method for analyzing toxic elements (Cd, Hg, Pb, and As) as well as other trace elements (Cr, Mn, Se, Ni, Cu, Zn, Mo, and Tl) in baby foods (pureed and cereal) using the Titan™ MPS microwave digestion system for sample digestion and the NexION 2000 ICP-MS equipped with a High Throughput System (HTS) for sample analysis, under US FDA EAM 4.7.
Experimental
Instrumentation
A Titan MPS microwave digestion system was used to digest the samples. All studies were carried out using a PerkinElmer Inc. NexION 2000 ICPMS with HTS and an S20 series autosampler.
HTS utilizes flow injection technology to deliver the sample to the plasma during the data acquisition stage and deflects it to the waste for the rest of the time, reducing both the sample’s time in the plasma and wash times, where it can perform the wash procedure more efficiently than traditional sample introduction.
Table 1 lists the instrument components and operating settings utilized in the analysis.
Table 1. NexION ICP-MS Instrument Components and Operating Conditions. Source: PerkinElmer Food Safety and Quality
Instrument Component |
Type/Value |
Nebulizer |
ST-PFA MicroFlow |
Spray chamber |
Quartz cyclonic |
Torch |
One-piece quartz torch, 2 mm injector |
Cones |
Nickel sampler and skimmer cones
Aluminum hyper-skimmer cone |
Peristaltic pump tubing |
Carrier/Internal standard: orange/green (0.38 mm i.d.)
Waste: gray/gray Santoprene (1.30 mm i.d.) |
Sample uptake rate |
0.2 mL/minute |
Operating Conditions |
Type/Value |
RF power |
1600 W |
Plasma gas flow |
15 L/minute |
Auxiliary gas flow |
1.2 L/minute |
Nebulizer gas flow |
Optimized for <2.5% oxides;
double charge < 2.5% |
Cell gas |
O2, He, NH3 |
Instrument Optimization and Method Development
The device was set for best sensitivity and oxide as well as doubly-charged ion ratios before sample analysis. It is worth noting that new or freshly cleaned cones must be conditioned before being optimized. The cones were conditioned using this procedure, which involved aspirating the digested meal sample solution and monitoring the internal standards until the signals stabilized.
Table 2 lists the studied elements, their isotopes, and the technique of analysis employed for each element in this approach. To address the Pb natural abundance fluctuation, three isotopes of Pb were measured and their intensities were added.
Table 2. Elements, Isotopes, and Modes of Analysis for Different Elements. Source: PerkinElmer Food Safety and Quality
Element |
Mass |
Mode |
Cr |
52 |
Helium Collision |
Mn |
55 |
Helium Collision |
Cr* |
52 |
Ammonia Reaction |
Mn* |
52 |
Ammonia Reaction |
Ni |
60 |
Helium Collision |
Cu |
63 |
Helium Collision |
Zn |
66 |
Helium Collision |
As |
91 |
Oxygen Reaction |
Se |
94 |
Oxygen Reaction |
Mo |
95 |
Helium Collision |
Cd |
111 |
Helium Collision |
Hg |
202 |
Helium Collision |
Tl |
205 |
Helium Collision |
Pb+ |
206+207+208 |
Helium Collision |
* Elements measured in Reaction mode with ammonia.
+ Sum of three isotopes to address the natural abundance variation.
Reagents and Calibration Standards
Throughout this study, ultrapure water (UPW) with resistivity ≥ 18.2 MΩ.cm and high purity acids were employed. The calibration standards were created by diluting ICP-MS grade single-element standards in a diluent composed of 5% HNO3 and 0.5% HCl and spiked with 200 ppb gold to improve Hg mobility. The calibration blank and carrier solution was both made using this diluent.
Table 3 shows the concentrations of the calibration standards. Standard 4 was utilized as a CCV (continuous calibration verification) standard as well. The initial calibration verification (ICV) stock source was a second multi-element standard with verified values of 10 ppm for the target elements (excluding Hg) and was diluted to 10 ppb using the same diluent.
Table 3. Concentrations of the Calibration Standards. Source: PerkinElmer Food Safety and Quality
Element (ppb) |
Standard 1 (ppb) |
Standard 2 (ppb) |
Standard 3 (ppb) |
Standard 4 (ppb) |
Standard 5 (ppb) |
Mn, Zn, Cu |
1 |
10 |
50 |
100 |
250 |
Cr, Ni, As, Se, Mo, Cd, Hg, Tl, Pb |
0.1 |
1 |
5 |
10 |
25 |
Hg |
0.01 |
0.1 |
0.5 |
1 |
2.5 |
The internal standard solution contained 400 ppb Sc, 20 ppb Rh, and 20 ppb Ir in a solution of 1% HNO3, 0.5%HCl, and 2% isopropanol (IPA).
To address the carbon-induced signal amplification effects on elements with high ionization potential, such as As and Se, isopropanol was used to compensate for the difference in carbon concentration between the standards and the samples.
The internal standard was inserted into the HTS valve’s allocated port and mixed with the sample in real-time. The wash solution was made up of 1.5% HCl (v/v) and 1.5% HNO3 (v/v) spiked with 200 ppb gold and 5% IPA, with the latter being employed to help wash away organic contaminants.
Sample Preparation
The accuracy of the approach for pureed baby foods and dry cereal goods was validated using a baby food composite standard reference material (SRM) 2838a and a rice flour SRM 1568b, both bought from the National Institute of Science and Technology.
To verify the accuracy and recoveries of the analytical method, one pureed baby food sample made of fruits and vegetables, namely Fruit Meal, and one dry baby cereal, namely Rice Cereal, were used to assess duplicity and equipped for fortified analytical portions (FAPs), in which the specimens were spiked with multi-element standards at two concentration levels before digestion, as shown in Table 4.
Table 4. Concentrations of Spikes in the Analytical Solutions of the FAPs. Source: PerkinElmer Food Safety and Quality
Element |
Low Spike Level (L1) (ppb) |
High Spike Level (L2) (ppb) |
Cr |
1 |
10 |
Ni |
1 |
10 |
As |
1 |
10 |
Se |
1 |
10 |
Mo |
1 |
10 |
Cd |
1 |
10 |
Pb |
1 |
10 |
Tl |
1 |
10 |
Hg |
0.1 |
1 |
Mn |
10 |
100 |
Cu |
10 |
100 |
Zn |
10 |
100 |
A few drops of ultrapure water (UPW) were used to pre-wet the digestive vessel. Baby food samples and SRMs (about 2 g pureed sample and 0.5 g dry sample) were weighed into 75 mL microwave digestion containers, then 1 mL UPW, 8 mL HNO3, 2 mL H2 O2, and 24 µL gold single-element standard (1000 ppm) were added.
As a Hg stabilizer, gold was added. The built-in technique for rice flour was used to digest the method blanks, SRMs, infant food samples, and FAPs in the same batch.
Table 5 lists the microwave digestion parameters. The digests were quantitatively transferred into pre-weighed 50 mL centrifuge tubes after digestion by decanting the samples into pre-weighed centrifuge tubes, washing the vessels twice with 10 mL of UPW, and adding these rinsings to their corresponding centrifuge tubes.
To make the second sample stock solution, 0.5 mL of high-purity concentrated HCl was added to the centrifuge tube, followed by UPW to the 40 mL mark, and the weight was recorded.
The second stock sample solution (16 mL) was pipetted into a 50 mL centrifuge tube that had been pre-weighed, with UPW added to the 40 mL mark, the weight recorded, and the sample examined by ICP-MS.
Table 5. Microwave Digestion Parameters. Source: PerkinElmer Food Safety and Quality
Step |
Target Temperature (°C) |
Pressure (Bar) |
Ramp Time (min) |
Hold Time (min) |
Power (%) |
1 |
160 |
30 |
5 |
5 |
90 |
2 |
190 |
30 |
3 |
20 |
100 |
3 |
50 |
30 |
1 |
15 |
0 |
Results and Discussion
As and Se Analysis using Reaction Mode with Oxygen
Standard solutions of 5 ppb As and Se in 3% HNO3 and 0.5% HCl were spiked with Zr and Mo at various concentrations up to 1000 ppb to investigate the possible isobaric interference of 91Zr+ and 94Mo+ on 75As 16O+ and 78Se 16O+, respectively.
Figure 1 shows that accurate As and Se recoveries were acquired for the entire spectrum tested, which is higher than the concentration of Zr and Mo that can be anticipated in normal food components, demonstrating that Zr and Mo do not interfere with As and Se analysis using Reaction mode under the experimental conditions assessed.
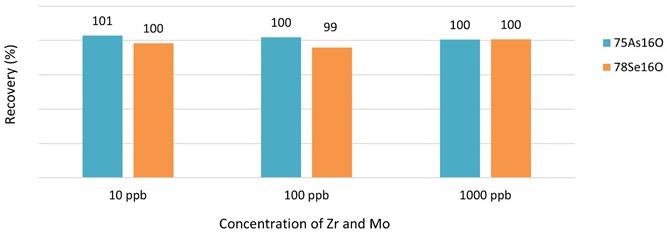
Figure 1. Recoveries of As and Se in the presence of Zr and Mo measured in Reaction mode with oxygen. Image Credit: PerkinElmer Food Safety and Quality
Detection Limits
The analytical solution detection limit (ASDL) and analytical solution quantification limit (ASQL) were computed using the standard deviation of 10 repeated measurements of the method blank, as stated in EAM 4.7 and EAM 3.26, respectively.
The ASDLs and ASQLs produced in this manner were substantially lower than those reported in EAM 4.7 for all 12 elements, frequently by more than an order of magnitude (Figure 2). In Reaction mode with NH3, the ASDLs and ASQLs for Cr and Mn were 10 and 5 times lower than in Collision mode with KED, respectively.
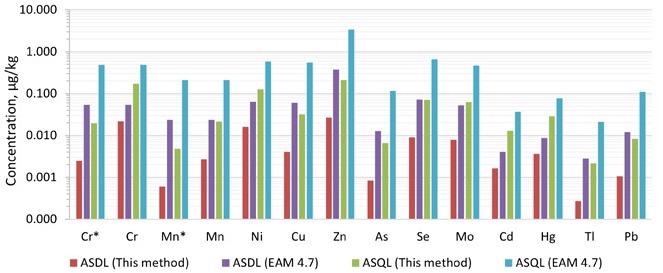
Figure 2. The analytical solution limits of this method and that provided in EAM 4.7. *Measured in ammonia Reaction mode. Image Credit: PerkinElmer Food Safety and Quality
By multiplying ASDL and ASQL with the dilution factor, the limit of detection (LOD) and limit of quantification (LOQ) were obtained. For the pureed infant food samples, the dilution factor was about 50, while for the cereal samples, it was around 200.
Figures 3 and 4 illustrate comparisons of the detection limits obtained in the technique with those provided in EAM 4.7 for each category, as well as the action levels for each of the dangerous components. The LODs and LOQs produced in the technique were lower than the nominal limitations established in EAM 4.7 for all 12 elements needed by the standard.
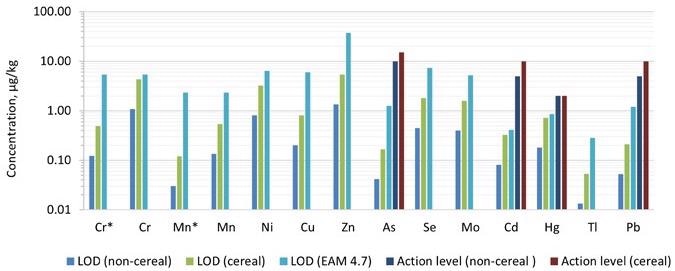
Figure 3. Comparison of limits of detection (LODs) achieved in this work compared with those captured in EAM 4.7. Dilution factors of 50 and 200 were applied for the pureed (non-cereal) baby food and the baby cereal, respectively. The action levels proposed in the “Baby Food Act 2021” are also plotted for comparison. *Measured in ammonia Reaction mode. Image Credit: PerkinElmer Food Safety and Quality
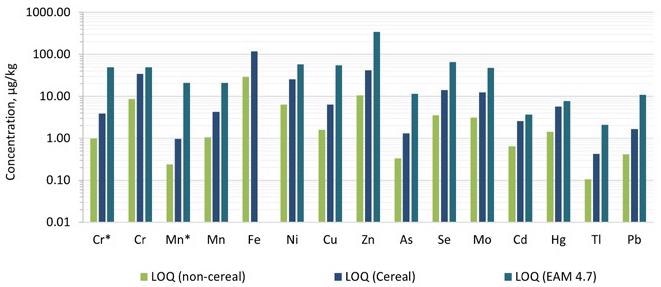
Figure 4. Comparison of limits of quantification (LOQs) achieved in this work compared with those captured in EAM 4.7. Dilution factors of 50 and 200 were applied for the pureed (non-cereal) baby food and the baby cereal, respectively. *Measured in ammonia Reaction mode. Image Credit: PerkinElmer Food Safety and Quality
Accuracy
The method’s accuracy was confirmed by comparing the recoveries of different analytes in SRM 2383a (Baby Food Composite), SRM 1568b (Rice Flour), and FAPs. The SRM was made in triplicates and measured three times each time.
Figure 5 shows the average recoveries of the triplicates. For the approved elements, recoveries of 90 to 110% were obtained (Figure 5), well within the QC standards of 80 to 120% outlined in EAM 4.7. Since the elements Cr, Ni, and Tl were not approved for the tested SRMs, they are not depicted in Figure 5.
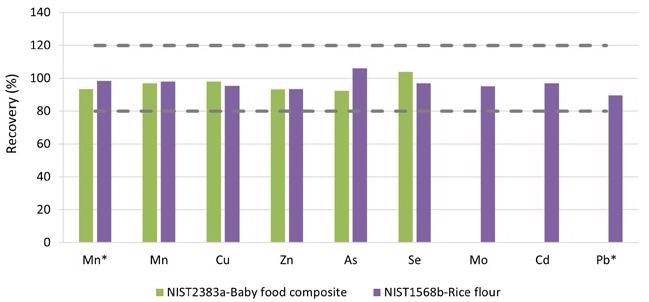
Figure 5. Recoveries for the certified values in SRM 2383a (Baby Food Composite) and SRM 1568b. *Measured in ammonia Reaction mode. Image Credit: PerkinElmer Food Safety and Quality
FAP tests were done at two spike levels, L1 and L2, on the pureed baby food “Fruit Meal” and the infant cereal “Rice Cereal,” as shown in Table 4. For each spiking sample, duplicates were made. Figure 6 depicts the average recoveries. All target components had recovery rates between 87% and 110%, which was well within the QC requirements of 80–120% outlined in EAM 4.7.
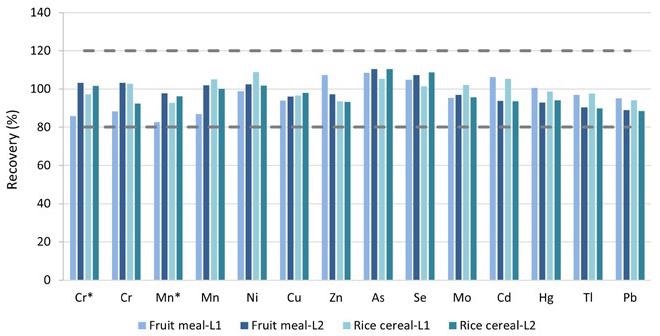
Figure 6. FAP test results on the pureed baby food (Fruit Meal) and the dry baby cereal (Rice Cereal) samples. *Measured in ammonia Reaction mode. Image Credit: PerkinElmer Food Safety and Quality
Precision
The relative percent difference (RPD) of repeated sample analysis was used to assess precision. Table 6 shows the results of RPD testing performed on two infant food samples bought. The RPDs for both samples were under the QC criterion of 20% for all target components, as indicated in EAM 4.7.
Table 6. RPD Test Results on the Pureed Baby Food (Fruit Meal) and Baby Cereal Samples. Source: PerkinElmer Food Safety and Quality
|
Fruit Meal |
Rice Cereal |
Element |
Measured Concentration
(μg/Kg) Portion A |
Measured Concentration
(μg/Kg) Portion B |
RPD (%) |
Measured Concentration
(μg/Kg) Portion A |
Measured Concentration
(μg/Kg) Portion B |
RPD (%) |
Cr* |
31.31 |
37.05 |
17% |
131.07 |
131.31 |
0.2% |
Cr |
31.33 |
36.46 |
15% |
128.77 |
128.36 |
0.3% |
Mn* |
3311.56 |
3378.80 |
2% |
10450.53 |
10789.83 |
3% |
Mn |
3579.49 |
3649.23 |
2% |
10825.25 |
11044.67 |
2% |
Ni |
137.02 |
137.86 |
1% |
239.76 |
249.15 |
4% |
Cu |
1482.91 |
1537.65 |
4% |
2032.26 |
2100.86 |
3% |
Zn |
1250.67 |
1279.91 |
2% |
19334.79 |
19434.17 |
1% |
As |
0.68 |
0.67 |
1% |
11.76 |
11.72 |
0.4% |
Se |
2.50 2.53 |
2.53 |
1% |
84.30 |
84.27 |
0.04% |
Mo |
10.72 |
10.91 |
2% |
154.14 |
151.63 |
2% |
Cd |
1.95 |
1.85 |
5% |
3.84 |
4.19 |
9% |
Hg |
0.24 |
0.23 |
5% |
<LOD |
<LOD |
-- |
Tl |
0.12 |
0.12 |
7% |
0.85 |
0.90 |
6% |
Pb |
1.37 |
1.39 |
1% |
<LOD |
<LOD |
-- |
*Measured in ammonia Reaction mode
Stability Check
Baby food samples were assessed regularly over 8 hours to confirm the method’s stability, and CCV recoveries were tracked throughout this time. All of the CCV recoveries were standardized to Standard 3 (Table 3) and, as shown in Figure 7, were well within 10% of the original value, fulfilling the QC requirement of 100 ± 10% as indicated in EAM 4.7.
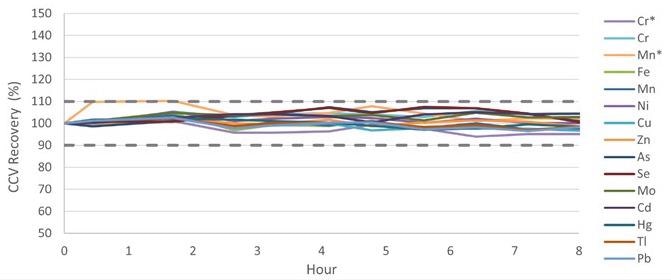
Figure 7. CCV recoveries over an 8-hour run of baby foods samples. *Measured in ammonia Reaction mode. Image Credit: PerkinElmer Food Safety and Quality
Conclusions
The NexION ICP-MS was used to prepare and analyze infant food samples from two categories, pureed and cereal, for reliable identification of harmful elements such as As, Cd, Hg, and Pb, as well as eight additional trace elements (Cr, Mn, Ni, Cu, Zn, Se, Mo, and Tl) specified in EAM 4.7.
- Spectral interferences were removed/mitigated using a variety of gas modes
- The infant food samples were digested using a built-in technique for this sample type on the Titan MPS microwave system
- Reaction mode with ammonia improved sensitivity and detection limits for Cr and Mn, resulting in greater performance when compared to Collision mode with KED, where method development was basic
- To boost sample throughput and shorten total sample residence time in the plasma, flow injection with HTS was employed for sample introduction
Following the EAM 4.7 method, the method was evaluated in terms of detection limits, accuracy, precision, and stability, and was found to meet or exceed the QC criteria in each category, with LODs lower than the action levels proposed in the “Baby Food Safety Act of 2021,” making the method and instrumentation suitable for routine analysis of baby foods and other related food and raw material samples.
References
- S.1019 - Baby Food Safety Act of 2021; https://www.congress.gov/bill/117th-congress/senate-bill
- U.S. FDA Closer to Zero: Action Plan for Baby Foods (8 April 2021); https://www.fda.gov/food/environmental-contaminants-food/closer-zero-reducing-childhood-exposure-contaminants-foods
- Gray J. P, et al. (2015) 4.7 Inductively Coupled Plasma-Mass Spectrometric Determination of Arsenic, Cadmium, Chromium, Lead, Mercury and Other Elements in Food Using Microwave Assisted Digestion, U.S. FDA Elemental Analysis Manual
- Larsen, H. E, et al. (1994) Carbon-enhanced Inductively Coupled Plasma Mass Spectrometric Detection of Arsenic and Selenium and Its Application to As Speciation; Journal of Analytical Atomic Spectrometry. doi:10.1039/JA9940901099
- Ariga, T, et al. (2019) Study on carbon-induced signal enhancement in inductively coupled plasma mass spectrometry: an approach from the spatial distribution of analyte signal intensities. Journal of Analytical Atomic Spectrometry. doi:10.1039/C9JA00152B
- Cunningham, C. W, et al. (2014) U.S. FDA Elemental Analysis Manual for Food and Related Products: 3.2. Terminology (September 2014).
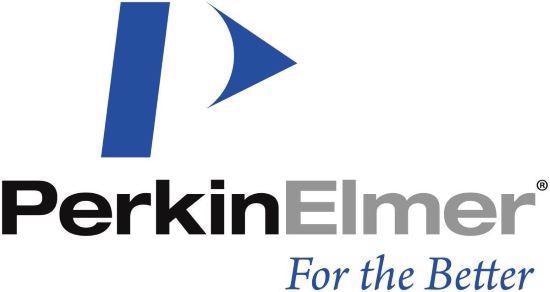
This information has been sourced, reviewed and adapted from materials provided by PerkinElmer Food Safety and Quality.
For more information on this source, please visit PerkinElmer Food Safety and Quality.