Engineering materials, such as metals, plastics, ceramics, and concrete, are susceptible to wear, aging, and damage from environmental factors. Inspired by nature, researchers are now looking at biological materials like biofilms, bone, skin, and bark as model systems to develop novel living materials that can grow, self-repair, and adapt to environmental changes.
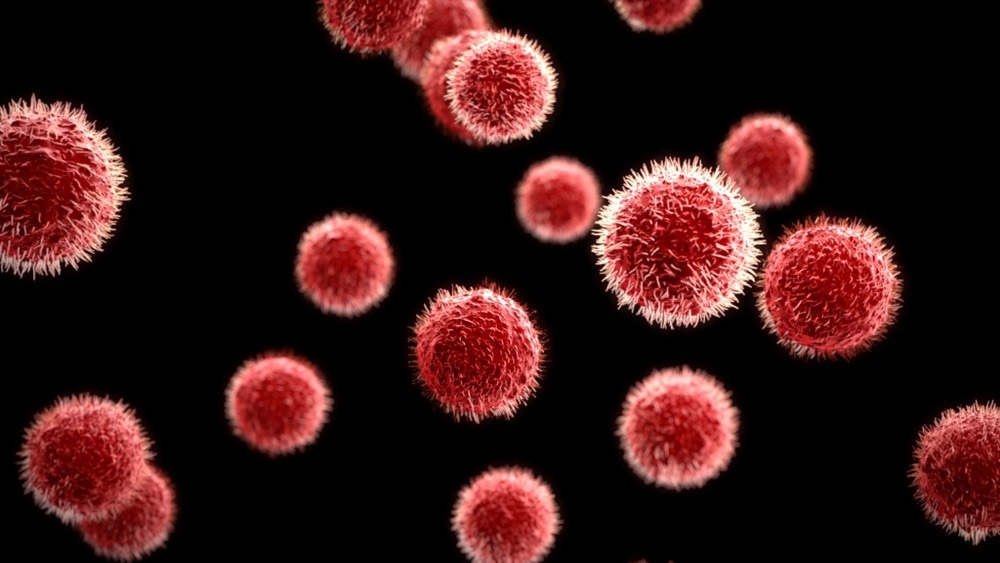
Image Credit: kridipol poolket/Shutterstock.com
Bacterial organisms have evolved to employ biopolymer-based materials, called biofilms, enabling them to form colonies and survive even in extreme environments. Such bacterial biofilms exhibit some fascinating properties, such as toughness, high surface adhesion, and water and oil resistance, that make it difficult to remove or inactivate them in settings like medicine and food manufacturing where the presence of bacteria is undesirable.
Inspired by the outstanding versatility and sturdiness of bacterial biofilms, material scientists are exploring ways of harnessing those properties and creating bacterial-based living materials suitable for a wide range of industrial applications, ranging from agriculture and construction to biotechnology and electrical engineering.
Biominerals as Smart Construction Materials
Researchers at the Department of Civil and Environmental Engineering at the University of Southern California, led by Prof. Qiming Wang, focused on bacteria-assisted mineralization processes combined with the additive manufacturing of polymer scaffolds to design mineralized composites with high fracture toughness. High-toughness biological mineral materials, such as teeth, pearl, and nacre, combine high mineral volume fractions with highly complex microstructures.
The researchers utilized a particular urease-producing (an enzyme that hydrolyzes urea) bacterial strain S. pasteurii. When urea and calcium ions are supplied to the bacterial colony, it forms calcium carbonate deposits, like the compounds in our bones and teeth. By using 3D-printed scaffolds to guide bacterial growth and calcium carbonate deposition, the scientists succeeded in creating microstructured mineralized composite materials.
The growth process enabled Prof. Wang's team to control the mineral volume-fraction in a wide range (45% -90%) while tuning the material's microarchitecture, thus ensuring exceptional fracture toughness and energy absorption capacity superior to both natural and artificial counterparts. Importantly, the material exhibits self-growing and self-repairing properties, which can be triggered by introducing a living bacterial culture to the damaged part.
Bacterial Biofilm Formation and Properties
Bacterial biofilms are sticky and slimy substances composed of extracellular biopolymers used by bacteria for surface attachment and protection from harsh environments. Depending on the bacterial strain and the growth conditions, the biofilm composition can vary, resulting in a wide range of mechanical and chemical properties.
From a materials science point of view, some of the biofilm's unique properties are highly desirable and offer a variety of possibilities for applications in biotechnology, medicine, and constriction. Of particular interest to scientists and engineers are the visco-elastic properties of the biofilms and their ability to efficiently repel different fluids, ranging from water to organic solvents, thus protecting the underlying substrate from corrosion and abrasion.
The properties of biofilms can be modified by manipulating their composition (adding polymers, nanoparticles, or small molecules), or by tailoring the biofilm structure.
Biofilm Tailoring Using Additive Manufacturing
Researchers from the Delft University of Technology in the Netherlands and the University of Rochester in the USA employed an innovative approach for the fabrication of biofilm-derived functional materials with the aid of 3D printing technology. The cost-efficient fabrication method enabled the scientist to print biofilms with submillimeter accuracy mimicking the structural heterogeneity of natural biofilms. By controlling factors like bacterial strain, biofilm composition, and the concentration of various additives, the researchers were able to tailor the functionality, rheological properties, and morphology of the printed biofilm.
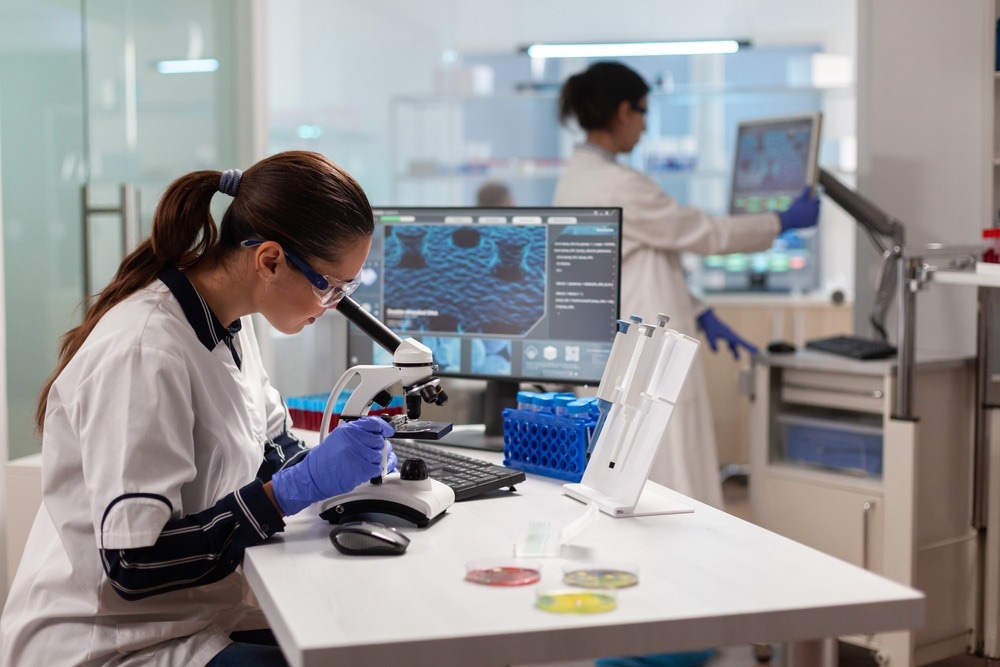
Image Credit: DC Studio/Shutterstock.com
The most recent achievement of the research collaboration was the development of 3D-printed living materials that incorporate bacteria capable of degrading biopolymers like polyhydroxybutyrate (a natural polyester produced by many bacterial strains), thus enabling precise control over when and how quickly the biopolymers degrade.
Further development of the approach aims to enable the additive manufacturing of living materials incorporating genetically engineered bacteria that can trigger bioplastic degradation upon demand at the product’s end-of-life.
Engineering Bacterial Biopolymers for Sustainable Applications
Biopolymers are synthesized by bacteria using enzymes that polymerize sugars, amino acids, or hydroxy fatty acids to yield biological macromolecules, such as polysaccharides, polyamides, polyesters, and others. Typically, the biopolymers are biodegradable and often can be used as obtained without downstream processing. Their industrial application depends on the type of microorganism source. Polysaccharides, like alginate, cellulose, carrageenan, and starch, have a wide range of commercial applications in the food and pharmaceutical industry, but there are other applications. The main drawback of these materials is their variable quality and lack of guaranteed supply.
In recent years, synthetic biology and bioengineering combined with cost-efficient polymer processing methods enabled the development of numerous novel high-value biodegradable polymers for different applications, including food packaging, automotive components, biomedical devices, and many others. The growing use of advanced biodegradable materials not only reduces the carbon footprint of a wide range of industrial processes but also reduces the environmental impact of a growing number of consumer products.
More from AZoM: How are Bioplastics Made?
References and Further Reading
Baily, S. (2022) Researchers Collaborate on Ocean-Degradable Bioplastics. [Online] AZoMaterials. Available at: https://www.azom.com/article.aspx?ArticleID=22112
Xin, A., et al. (2021) Growing Living Composites with Ordered Microstructures and Exceptional Mechanical Properties. Adv. Mater., 33, 2006946. Available at: https://doi.org/10.1002/adma.202006946
Qiu, J, et al. (2021) Engineering living building materials for enhanced bacterial viability and mechanical properties. iScience, 24(2), 102083. Available at: https://doi.org/10.1016/j.isci.2021.102083
Baily, S. (2021) Producing Biofilms with Additive Manufacturing. [Online] AZoMaterials. Available at: https://www.azom.com/article.aspx?ArticleID=21084
Moradali, M.F., Rehm, B.H.A. (2020) Bacterial biopolymers: from pathogenesis to advanced materials. Nat Rev Microbiol 18, 195–210. Available at: https://doi.org/10.1038/s41579-019-0313-3
Balasubramanian, S., et al. (2019) 3D Printing for the Fabrication of Biofilm-Based Functional Living Materials. ACS Synthetic Biology, 8 (7), 1564-1567. Available at: https://doi.org/10.1021/acssynbio.9b00192
Disclaimer: The views expressed here are those of the author expressed in their private capacity and do not necessarily represent the views of AZoM.com Limited T/A AZoNetwork the owner and operator of this website. This disclaimer forms part of the Terms and conditions of use of this website.