Sponsored by Gatan, Inc.Reviewed by Olivia FrostFeb 8 2023
There is increasing demand for high-performance lithium-ion batteries for energy storage, electric vehicles, and electronic devices. One of the most commonly used cathode materials in these batteries is LiNixMnyCozO2, also known as NMC.
NMC is employed in the form of polycrystalline particles, with the orientation, grain size, and grain boundary structure within each particle having a significant impact on the charging-discharging properties and degradation behavior of the battery cell.
To study the crystallographic microstructure of these materials, Electron Backscatter Diffraction (EBSD) is considered the most suitable microanalysis technique when used in conjunction with a Scanning Electron Microscope (SEM).
However, due to the small grain size of NMC crystals and stringent sample preparation requirements, achieving satisfactory resolution of the grains using traditional EBSD analytical conditions can prove challenging.
This article outlines how the Clarity™ Direct Detector was employed in a study to collect data at lower beam energies and currents, resulting in improved EBSD spatial resolutions.
Results and Discussion
A cross-section of an NMC cathode layer was prepared using a low-energy broad ion beam source. This approach produces a pristine surface with high-quality EBSD patterns.
In an ideal world, the lithium-ion battery specimen would be instantly transferred to an SEM using a vacuum exchange solution, such as the PECS™ II System from Gatan.
However, in this case, the sample was shipped for analysis and exposed to the atmosphere for an extended period before EBSD analysis, resulting in the degradation of the sample surface.
The EDAX Clarity EBSD Analysis System with APEX™ 2.0 Software was used to gather EBSD data. The diffracted electrons are captured directly onto a pixelated sensor, removing the requirement for a phosphor screen and optical connection, resulting in increased sensitivity and performance at lower beam energy and currents.
Figure 1a depicts an EBSD Inverse Pole Figure (IPF) orientation map recorded at 20 kV acceleration potential, 1.6 nA beam current, and 45 patterns per second acquisition frequency.
The colors correspond to the crystallographic orientations aligned with the sample's normal direction, using the colored IPF triangle as a key. The black areas in this map correspond to points with a low confidence index, indicating that the acquired EBSD patterns cannot be reliably analyzed.
The majority of these points correspond to the binding substance utilized in the cathode layer. However, there are considerable dark patches between measured grains within the particle for the large NMC polycrystalline particle in the center of the figure.
This is a result of the size of the interaction volume at this acceleration voltage spreading across multiple grains when acquiring data near grain boundaries, coupled with the degradation of EBSD pattern quality due to exposure. It is worth noting that the index of the single-crystal particle is far more dependable.
Figure 1b illustrates an IPF map that was collected at the same rate as the previous figure but from a different particle while reducing the beam dose to 10 kV energy and 400 pA current.
This reduction in beam dose improves the spatial resolution within the polycrystalline NMC particle as well as the microstructural characterization, allowing for a better correlation of grain boundary structure with degradation behavior.
Moreover, it achieves this enhanced spatial resolution without the need to create an electron beam transparent sample for Transmission Kikuchi Diffraction (TKD). Additionally, the lower beam doses also minimize charging effects, which is crucial for samples with non-conductive binder material.
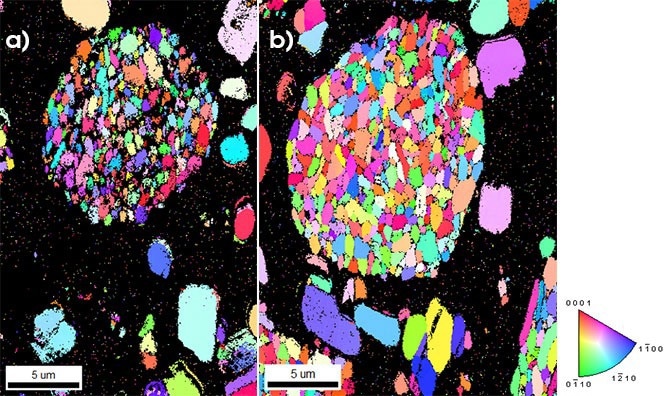
Figure 1. EBSD IPF orientation maps of NMC cathode particles used in lithium-ion batteries were obtained using the Clarity Direct Detector. For Figure 1a, the data was collected at 20 kV acceleration voltage and 1.6 nA beam current. The black points represent measurements that could not be analyzed with high confidence. For Figure 1b, the data was collected at 10 kV acceleration voltage and 400 pA beam current. The characterization of the grains within the primary particle is much better. No data cleanup routines were used for these figures. Image Credit: EDAX.
In the examples provided, no data clean-up routines were employed, and only the IPF coloring was shown. However, the indexing performance at grain boundaries can be improved by combining the IPF map with an EBSD image or pattern-quality greyscale map.
Due to overlapping patterns, the image quality (IQ) value at grain boundaries diminishes, and hence the deeper coloring of the IQ value conceals the lower confidence indexing. It is critical to understand how these combined contrasts influence data interpretation, especially when assessing indexing performance.
Conclusion
The use of the Clarity Direct Detector at lower electron beam energies and currents offers an advantage by reducing the beam interaction volume and increasing EBSD spatial resolution. This allows for better characterization of the NMC cathode materials used in lithium-ion batteries and improves the understanding and performance of these materials.
References
- Quinn et al. (2020) Electron Backscatter Diffraction for Investigating Lithium-Ion Electrode Particle Architectures. Cell Reports Physical Science 1,100137.
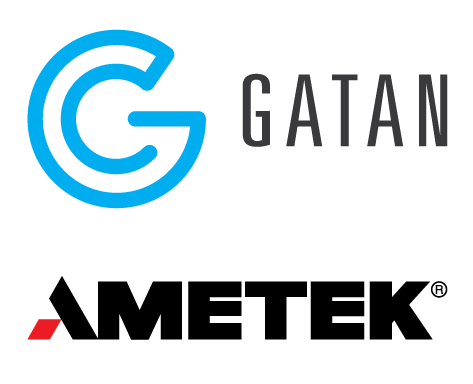
This information has been sourced, reviewed and adapted from materials provided by Gatan Inc.
For more information on this source, please visit Gatan Inc.