This article discusses the role of salts, specifically lithium salts, in electrolytes to reduce the flammability of lithium-ion batteries.
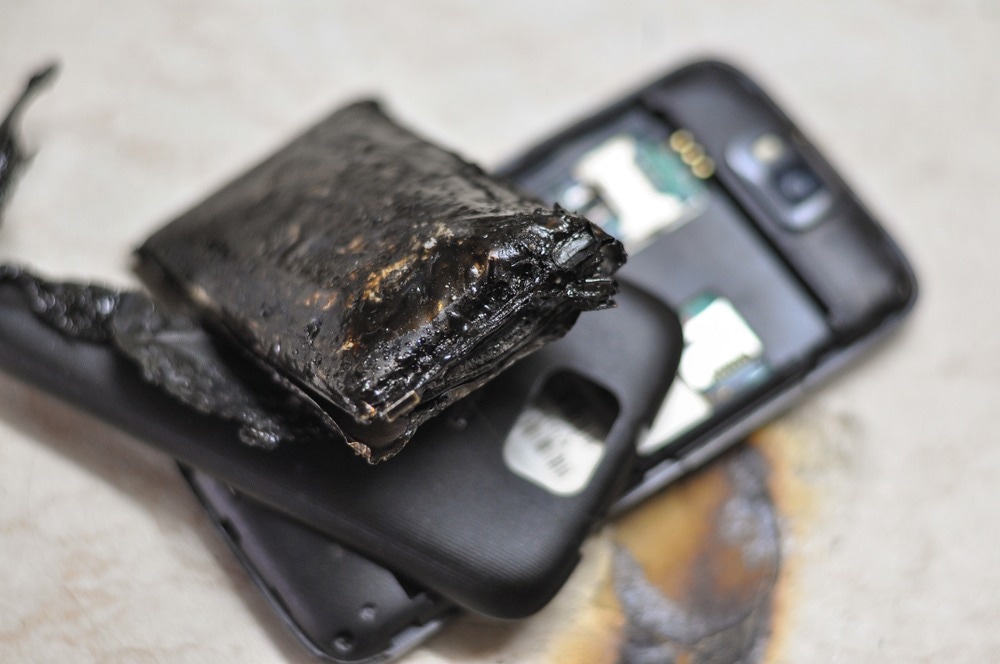
Image Credit: Ivan Marjanovic/Shutterstock.com
Why are Lithium-ion Batteries Flammable and Prone to Explosions?
Lithium-ion batteries are used extensively in grid energy storage, portable devices, and electric vehicles owing to their stable cycling performance and high specific energy densities.
For instance, advanced lithium-ion batteries possess a specific energy of 250 Wh kg−1, while lithium-air and lithium-sulfur batteries possess a specific energy of 950 Wh kg−1 and 650 Wh kg−1, respectively.
However, chemical energy in lithium-ion batteries can be released abruptly in the form of explosions or fires when the batteries are operated improperly. Additionally, the electrolyte also plays a crucial role in increasing the flammability of lithium-ion batteries.
Electrolytes based on the mixtures of cyclic and linear carbonates and lithium hexafluorophosphate (LiPF6) are commonly used in lithium-ion batteries. Linear carbonates, such as diethyl carbonate (DEC), ethyl methyl carbonate (EMC), or dimethyl carbonate (DMC), are used lower the electrolyte viscosity and increase electrolyte conductivity.
However, cyclic and linear carbonates are highly flammable and volatile and have flash points between 16 oC and 33 oC. Thus, these carbonates can cause explosions and fires when they are combined with an ignition source and an oxidant.
Thermal runaway is one of the significant risks in lithium-ion batteries that occurs due to an uncontrolled exothermic chemical reaction triggered by high temperatures during operation.
The rate of exothermic chemical reaction increases when the battery temperature rises above 80 oC, which further heats up the battery, leading to a positive feedback cycle. Eventually, the continuously rising temperatures lead to fire/smoke/explosions or violent cell venting.
Overcharging of the lithium-ion battery due to an inefficiently designed battery management system (BMS) or incompatibility between the charger and the cell, exposure of the battery to extremely low or high temperatures, external short circuits due to faulty wiring, and internal shorting are primarily responsible for the initial overheating, which later leads to the thermal runaway.
Frequent lithium-ion battery explosions and fires have caused several accidents involving electric vehicles, laptops, and cell phones, leading to the loss of lives in certain cases. These incidents have increased the need to reduce the flammability of lithium-ion batteries to use them safely in future applications based on high-energy battery systems.
Ways to Mitigate the Fire Hazard in Lithium-ion Batteries and their Limitations
The use of flame-retardant electrolyte additives or non-flammable electrolyte solvents can reduce the flammability and mitigate the fire hazard in lithium-ion batteries. Specifically, flame-retardant additives can effectively improve the safety of flammable electrolytes.
However, these additives can adversely impact the electrochemical performance of the batteries, such as by reducing the cycling stability. Similarly, solvent-free polymer electrolytes possess insufficient ionic conductivity for room-temperature battery operation.
Feasibility of Using Salts to Reduce Lithium-ion Battery Flammability
In a study recently published in the journal Matter, researchers synthesized a liquid-state polymer electrolyte with a higher amount of lithium salt to make flameproof lithium-ion batteries with a good electrochemical performance at room temperature.
The electrolyte, called solvent-anchored non-flammable electrolyte (SAFE), was composed of flammable dimethoxyethane (DME) solvents, lithium bis(fluorosulfonyl)imide (LiFSI) salts, and polysiloxane tethered with ion-solvating moieties.
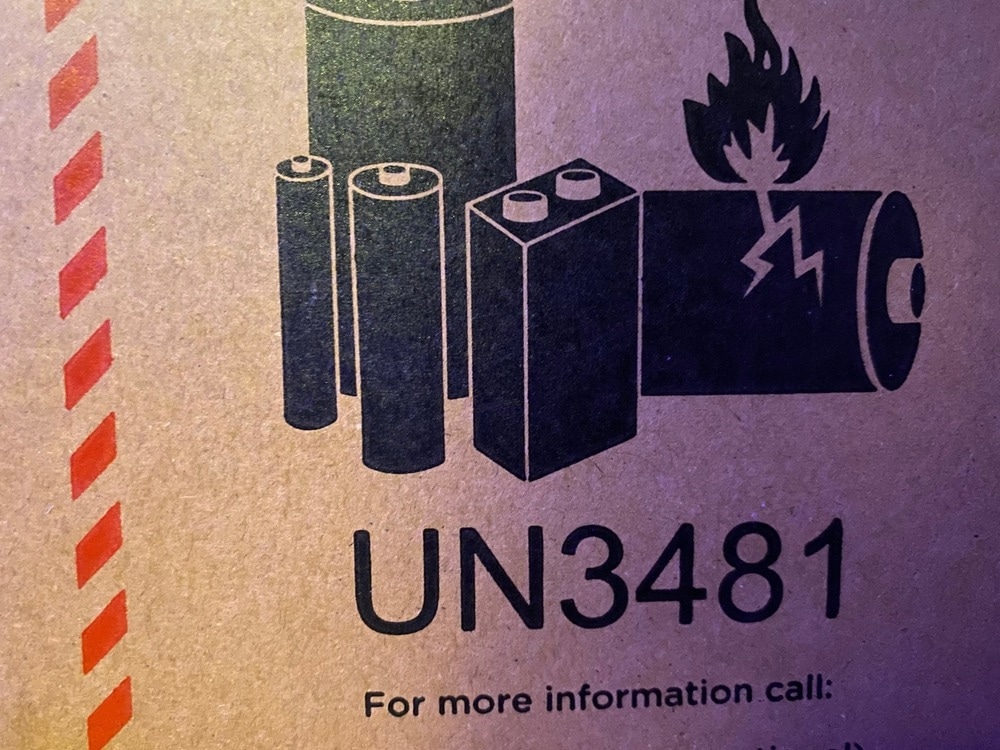
Image Credit: James Spindler/Shutterstock.com
Typically, salts constitute less than 50% of the weight of polymer-based electrolytes. However, the LiFSI salt in SAFE constituted 63% of the electrolyte weight, making SAFE one of the saltiest polymer-based electrolytes. Solvent molecules were anchored with the polymer and the salt to increase the ionic conductivity of the electrolyte without affecting its non-flammability.
Researchers investigated the feasibility of using the SAFE in lithium-ion batteries by pairing the electrolyte with commercially available electrodes. The non-flammable electrolyte demonstrated 1.6 mS/cm ionic conductivity at 25 oC/room temperature.
Additionally, the battery containing SAFE displayed superior cycling stability up to 400 cycles without any notable decline in capacity at room temperature and was operated safely in the 25 °C to 100 °C temperature range, which is higher than the current acceptable operating temperature range of −20 °C to 60 °C for lithium-ion batteries, despite containing a flammable solvent.
The higher amount of salt that acted as anchors for DME molecules prevented their evaporation, which mitigated the fire hazard in lithium-ion batteries. Both DME and LiFSI salts synergistically plasticized the polymer to improve ionic conductivity.
Thus, SAFE can effectively address the issue of flammability in lithium-ion batteries due to the use of intrinsically flammable electrolytes while ensuring good electrochemical performance. Moreover, SAFE can be paired with all commercially available electrodes without cell engineering due to its liquid nature.
To summarize, the effectiveness of SAFE in significantly increasing the safe operating temperature range of lithium-ion batteries has demonstrated the feasibility of adding salts in greater proportion to electrolytes to reduce the flammability of batteries at high temperatures, facilitating the development of safe batteries with high energy density.
More from AZoM: Advantages and Limitations of Zinc Carbon Batteries
References and Further Reading
Liu, K., Liu, Y., Lin, D., Pei, A., Cui, Y. (2018). Materials for lithium-ion battery safety. Science Advances, 4, 6. https://www.science.org/doi/10.1126/sciadv.aas9820
Huang, Z., Lai, J.-C., Kong, X., Rajkovic, I., Xiao, X., Celik, H., Yan, H., Gong, H., Rudnicki, P. E., Lin, Y., Ye, Y., Li, Y., Chen, Y., Gao, X., Jiang, Y., Choudhury, S., Qin, J., Tok, J. B. H., Cui, Y., Bao, Z. (2022). A solvent-anchored non-flammable electrolyte. Matter. https://doi.org/10.1016/j.matt.2022.11.003
Patrick, C. (2022) Flameproofing lithium-ion batteries with salt [Online] Available at https://engineering.stanford.edu/magazine/flameproofing-lithium-ion-batteries-salt (Accessed on 18 January 2023)
What Causes Thermal Runaway? [Online] Available at https://ul.org/research/electrochemical-safety/getting-started-electrochemical-safety/what-causes-thermal (Accessed on 18 January 2023)
Ma, S., Jiang, M., Tao, P., Song, C., Wu, J., Wang, J., Deng, T., Shang, W. (2018). Temperature effect and thermal impact in lithium-ion batteries: A review. Progress in Natural Science: Materials International, 28(6), 653-666. https://doi.org/10.1016/j.pnsc.2018.11.002
Hess, S., Wohlfahrt-Mehrens, M., Wachtler, M. (2015). Flammability of Li-Ion Battery Electrolytes: Flash Point and Self-Extinguishing Time Measurements. Journal of The Electrochemical Society. https://www.researchgate.net/publication/273105919_Flammability_of_Li-Ion_Battery_Electrolytes_Flash_Point_and_Self-Extinguishing_Time_Measurement
Disclaimer: The views expressed here are those of the author expressed in their private capacity and do not necessarily represent the views of AZoM.com Limited T/A AZoNetwork the owner and operator of this website. This disclaimer forms part of the Terms and conditions of use of this website.