Advanced materials are a class of innovative substances deliberately engineered to possess superior properties, enabling them to deliver exceptional performance. The advancements in materials science and engineering, combined with a deeper understanding of atomic structures, have laid the foundation for the emergence of advanced materials. This field encompasses a wide range of disciplines and applications, encompassing any newly engineered material or a novel iteration of an existing one that falls within the realm of advanced materials.
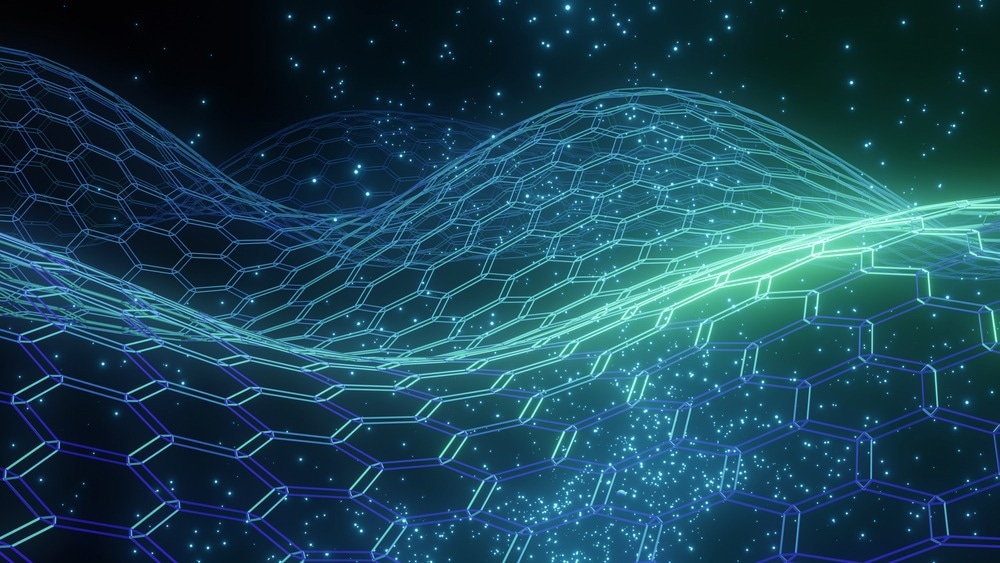
Image Credit: satoshi takahata/Shutterstock.com
Metal-Organic Framework Super-capacitors: The Future of Energy Storage
Clean energy storage technologies are playing a crucial role in reshaping the transportation sector, which has long been dominated by fossil fuels. They are also instrumental in efficiently storing energy generated from intermittent renewable sources. Among these technologies, super-capacitors have garnered considerable attention due to their ability to charge and discharge rapidly and their extended operational lifespan.
When it comes to selecting electrode materials for super-capacitors, certain criteria must be met. These criteria include high electrical conductivity, a superior surface area to facilitate efficient charge storage, and robust electrochemical stability. Traditionally, porous carbons have been the preferred choice for electrode materials. However, recent breakthroughs in research have piloted a transformative era in the development of super-capacitors.
The latest article published in Advanced Functional Materials has highlighted the importance of metal-organic frameworks (MOFs) for super-capacitors. MOFs, short for modular hybrid materials, showcase an extraordinary level of adaptability when it comes to their crystal structure, morphology, and chemical composition. This inherent flexibility results in a broad spectrum of physical properties that can be achieved within this class of materials.
The categorization of MOFs can be established based on the interconnectivity between their building blocks within their crystal structures, ranging from 1D chains to layered 2D sheets and intricate 3D networks.
The remarkable features of metal-organic frameworks (MOFs) have captured substantial interest and led to extensive research endeavors across a multitude of fields, including but not limited to molecule sieving membranes, gas storage, photo-catalysis, and photovoltaic applications. Additionally, MOFs have made significant strides in their application and advancement within electrochemical systems, spanning areas such as super-capacitors, batteries, and electro-catalysts.
Within the past decade, conductive MOFs have shown promising results; however, in comparison to porous carbon, there are several limitations that have been discovered. Presently, MOF systems are characterized by a noticeably narrower stable voltage window, typically falling within the range of 1 to 1.5 V. This is in contrast to the broader voltage range of approximately 2.5–2.7 V observed in materials like YP-50F porous carbon. Furthermore, an issue with existing MOF systems is their relatively lower rate or kinetic performance when compared to carbon-based electrode materials.
It's important to highlight that while these limitations may raise initial concerns, it's encouraging to note that extensive global research efforts are currently in progress to tackle and alleviate these challenges.
Novel Phase Change Memory Materials and Their Applications
In recent years, the demand for data storage has seen an exponential surge, garnering substantial attention when compared to other memory technologies. At the core of phase change memory technology are phase change materials (PCM), which play a crucial role in this context. The latest information on phase change memory materials has been discussed by researchers from China in the latest article published in Science and Technology of Advanced Materials.
Phase-change random-access Memory (PCRAM), as a non-volatile memory solution, possesses impressive performance and scalability. Its operation can be as fast as nanoseconds, solidifying its status as a prominent contender among the emerging nonvolatile memory technologies for next-generation electronic devices. In the domain of PCRAM, the critical role played by phase change materials highlights the utmost importance of in-depth research into the microstructure characterization and device applications of these materials.
The development and design of low-power phase-change memory (PCM) materials featuring conductive bridges represent effective tools for optimizing memory performance. A specific type of PCM, characterized by a mesh-like amorphous structure, has been engineered, boasting an impressively low power consumption rate of < 0.05 pJ. This energy consumption is a striking 1000 times lower than that of conventional products.
In this innovative approach, 'Nano-bridges' play a pivotal role by interconnecting crystalline domains to create a conductive channel. These 'Nano-bridges' are deliberately fractured to confine the subsequent phase-change switching within the region where the crystalline Nano-domain meets its amorphous surroundings. It's worth noting that this novel PCM and its fabrication process seamlessly integrate with existing 3D integration technology without incurring additional costs or requiring extra processing steps.
Phase-change materials (PCMs) are of great significance in computer memory systems because they can store data by changing their physical states. They can swiftly transition from a glassy to a crystalline state and retain that configuration for extended periods at lower temperatures when subjected to a thermal pulse.
Despite their widespread use in memory technology, driven by the temperature-dependent kinetics of their crystallization process, there has been an ongoing debate regarding the atomic-level transition mechanisms of certain PCMs in their amorphous phase. These mechanisms remain to be fully understood despite their utility in memory technology.
Progress in Laser-Based DED of Advanced Materials
Additive manufacturing (AM), often known as three-dimensional (3D) printing, allows for the rapid and on-demand fabrication of near-net-shape components directly from a computer-aided design (CAD) file. This eliminates the requirement for specialized, part-specific tooling.
Among the diverse branches of additive manufacturing, Directed Energy Deposition (DED) has emerged as a critical sector. The latest article in Materials Science & Engineering A has stated that DED has found widespread utility in the research and production of cutting-edge advanced materials, including a broad spectrum of substances such as advanced metals, ceramics, and composites.
Among the various metal Directed Energy Deposition (DED) processes, the most extensively researched method employs powder-based feedstock with a laser beam as the energy source. This technique, known as Laser Cladding (LC), is utilized to apply coatings onto the surfaces of advanced metallic materials. DED AM is often referred to as "3D laser cladding" since its origins trace back to a robotic multilayer cladding process.
In the context of cladding, DED involves the introduction of powder or wire into a molten pool generated by an energy source, such as a laser or electron beam, as it traverses the designated surface. During this procedure, thin layers of metallic or composite materials are deposited and fused onto a metallic component. This serves to enhance wear and corrosion resistance, optimize performance, or replace worn-out materials.
Implementation of Artificial Intelligence in Advanced Materials
Researchers at the Max-Planck-Institut für Eisenforschung (MPIE) have implemented a Machine learning algorithm for the development of corrosion-resistant alloy. Developing a single algorithm that accurately predicts corrosion behavior along with suggesting optimal alloy compositions is a major challenge. The implementation of AI has improved the predictive efficiency by at least 15% as compared to the existing tools.
Initially designed to address the critical issue of pitting corrosion in high-strength alloys, the versatility of the model extends to a broader range of alloy properties.
Current machine learning models primarily rely on numerical data, often overlooking the valuable insights contained within textual descriptors. Particularly in the context of explaining corrosion, critical information such as processing methodologies and experimental testing protocols is frequently conveyed through text.
In response to this, the research team ingeniously incorporated language processing methods into machine learning techniques designed for numerical data. The outcome is a comprehensive and fully automated natural language processing framework, effectively bridging the divide between textual and numerical information. This approach facilitates a more holistic understanding of corrosion phenomena by harnessing the power of both data types.
The field of advanced materials is progressing, with new materials being fabricated for specialized functionalities. The implementation of modern tools will surely boost performance and lead to a boost in applications of such materials. However, much focus is still needed to completely understand the working of a wide variety of advanced materials.
Semiconductor Membrane Fuel Cells: Pioneering Renewable Energy Technology
References and Further Reading
ICL, 2023. What are Advanced Materials?. [Online]
Available at: https://www.icl-group.com/blog/what-are-advanced-materials-industrial-applications/
Shin et. al (2023). "Metal-Organic Framework Supercapacitors: Challenges and Opportunities." Advanced Functional Materials. 2308479. Available at: https://doi.org/10.1002/adfm.202308497
Svetlizky. et. al. (2022). Laser-based directed energy deposition (DED-LB) of advanced materials. Materials Science and Engineering: A, 840, 142967. Available at: https://doi.org/10.1016/j.msea.2022.142967
Max-Planck-Institut für Eisenforschung, 2023. Artificial intelligence designs advanced materials. [Online]
Available at: https://www.mpie.de/4891546/ml-for-corrosion-science
Jiang et. al. (2023). "Microstructure characterization, phase transition, and device application of phase-change memory materials." Science and Technology of Advanced Materials. 2252725. Available at: https://doi.org/10.1080/14686996.2023.2252725
Disclaimer: The views expressed here are those of the author expressed in their private capacity and do not necessarily represent the views of AZoM.com Limited T/A AZoNetwork the owner and operator of this website. This disclaimer forms part of the Terms and conditions of use of this website.