Ceramic electrolytes in all-solid-state batteries have gained significant attention as the next-generation power source. Researchers are particularly interested in solid-state batteries due to their ability to overcome the defects and issues in traditional lithium-ion batteries. Moreover, they have distinctive properties such as high energy density, high ionic conductivity, chemical stability, non-flammability, and high mechanical strength.
The development of solid electrolytes, also known as ion-conductive ceramics, has been intensively researched, driven by the serious safety concerns associated with liquid electrolytes, such as electrolyte leakage and evaporation.
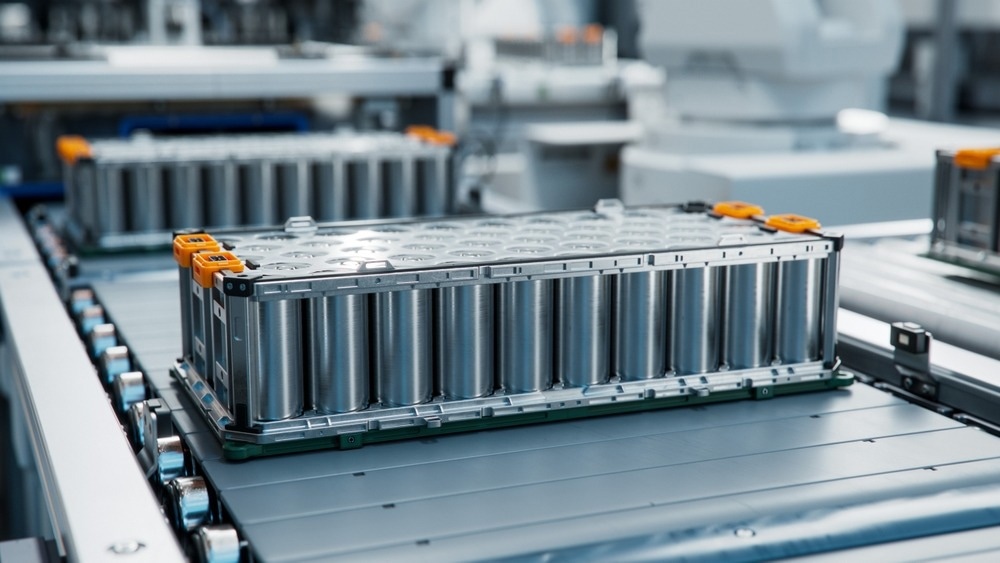
Image Credit: IM Imagery/Shutterstock.com
Ceramic Materials
Ceramic materials are inorganic, non-metallic solids composed of metal, non-metal, and metalloid atoms bound by covalent or ionic bonds. They have a range of structures, from crystalline and semi-crystalline to amorphous (glass), based on their structural ordering. Ceramic materials derive their properties from crystal structure and chemical composition.
Ceramic Electrolytes in Solid-State Batteries
Ceramic electrolytes eliminate the risks associated with leakage and evaporation common in liquid electrolytes. Most ceramics have limited ion migration due to a rigid skeleton structure. However, some ceramics allow efficient ion movement, resulting in high ionic conductivity comparable to liquid electrolytes.
These ceramics, known as "ion conductive ceramics," have garnered attention for various electrochemical applications, including power generation (solid oxide fuel cells, SOFC), energy storage such as batteries and capacitors, and atomic switches. Intensive research focuses on enhancing properties, especially ionic conductivity. When applied in electrochemical devices, they are termed "solid electrolytes." The use of ion-conductive ceramics in energy storage devices, such as batteries, has gained significant interest across research groups.
Ion species, such as O2−, Na+, and Li+, have been identified to flow in ceramics based on their structure and temperature. Ion-conductive ceramics with structures like fluorite, perovskite, apatite, K2NiF4, and AgI have garnered attention. While AgI and Rb4Cu16I7Cl13 have demonstrated high ionic conductivity, their widespread application has been limited by cost considerations and intricate preparation processes. Alternatively, ceramics conducting O2−, Na+, and Li+ have gained prominence due to their high ion conductivities, cost-effectiveness, and applicability in various electrochemical devices.
Fabrication Techniques for Ceramic Electrolytes in Batteries
The ceramic electrolyte is prepared by dry-pressing followed by high-temperature sintering. Dry pressing involves fine-milled powder premixed with approximately 5 wt % polyvinyl alcohol.
Alternatively, a more advanced colloidal process is employed for ceramic electrolyte preparation, ensuring not only high relative density but also uniform composition. Here, the key lies in creating suspensions with over 50 vol% solid loading and low viscosity (<1 Pa⋅s at a shear rate of 100 s–1), crucial for achieving non-porous casting and dense ceramics. It's noteworthy that careful solvent selection plays a vital role in preventing unwanted component diffusion or reactions within ceramic electrolytes.
Structural Diversity of Ion Conductive Ceramics
In ion-conductive ceramics, there are various structures, and each type affects performance differently. Structures like fluorite, perovskite, apatite, K2NiF4-type, and AgI are well-known as ion-conductive ceramics, with the fluorite structure characterized by stable ion conductivity, while perovskite structures contribute to high ionic conductivity. Despite the high ionic conductivity of AgI and Rb4Cu16I7Cl13, their practical application is limited due to cost considerations and intricate preparation processes. Given the challenges posed by some ceramics with high ionic conductivity, O2−, Na+, and Li+-conductive ceramics have received more attention for application in electrochemical devices due to their high ion conductivity and low production costs.
Applications of Ceramic Electrolytes in Solid-State Batteries
Applications of ceramic electrolytes in solid-state batteries cover various industries. Ceramic electrolytes in solid-state batteries are expected to be applied in many industries, especially in electric vehicles, due to their properties enhancing vehicle performance, such as longer driving ranges and shorter charging times. Furthermore, their importance extends to electronics applications, including smartphones and laptops, where high safety levels, energy density, and charging speed are crucial requirements.
Challenges in Processing and Environmental Impact
Despite the vast potential of ceramic electrolytes, there are some challenges, particularly in their manufacturing. High-temperature treatments, a common requirement in the synthesis of these materials, raise concerns about the environmental and economic feasibility of large-scale production. Life cycle analysis (LCA) becomes imperative to assess the overall environmental impact of batteries utilizing ceramic electrolytes. This involves considering factors such as energy consumption, emissions, and resource depletion throughout the entire life cycle of the batteries.
Conclusion
Ceramic electrolytes represent a groundbreaking advancement in all-solid-state batteries. Providing solutions to safety issues in traditional lithium-ion batteries, they exhibit properties such as high energy density, chemical stability, and non-flammability. Their ion-conductive nature ensures efficient ion movement, comparable to liquid electrolytes. Diverse structures like fluorite, perovskite, apatite, K2NiF4, and AgI contribute to the varied performance characteristics of ion-conductive ceramics.
However, there are some challenges in their manufacturing, primarily associated with high-temperature treatments, raising environmental and economic considerations for large-scale production. Life cycle analysis becomes crucial for evaluating the overall environmental impact, incorporating factors such as energy consumption and emissions throughout the batteries' life cycle. Despite these challenges, the ongoing pursuit of ceramic electrolytes reflects a commitment to innovation and safety in energy storage technologies, promising transformative applications across industries for enhanced performance and sustainability.
More from AZoM: Mechanical Properties of Steel in High-Stress Environments
References and Further Reading
Schreiber, A., Rosen, M., Waetzig, K., Nikolowski, K., Schiffmann, N., Wiggers, H., Küpers, M., Fattakhova-Rohlfing, D., Kuckshinrichs, W., Guillon, O., & Finsterbusch, M. (2023). Oxide ceramic electrolytes for all-solid-state lithium batteries – cost-cutting cell design and environmental impact. Green Chemistry. [Online] Available at: https://pubs.rsc.org/en/content/articlelanding/2023/gc/d2gc03368b
Ceramic Electrolytes for All-Solid-State Li Batteries. [Online] Available at: https://www.worldscientific.com/doi/pdf/10.1142/9789813233898_0001
Chen, A., Qu, C., Shi, Y., & Shi, F. (2020). Manufacturing Strategies for Solid Electrolyte in Batteries. Frontiers in Energy Research, 8, 2020. [Online] Available at: https://www.frontiersin.org/articles/10.3389/fenrg.2020.571440/full
Disclaimer: The views expressed here are those of the author expressed in their private capacity and do not necessarily represent the views of AZoM.com Limited T/A AZoNetwork the owner and operator of this website. This disclaimer forms part of the Terms and conditions of use of this website.