Humans have used fermentation to produce wine, bread, and other related products for centuries. An article published in Microorganisms describes fermentation as the conversion of sugars or carbohydrates into alcohols or acids, which may be used for human consumption.
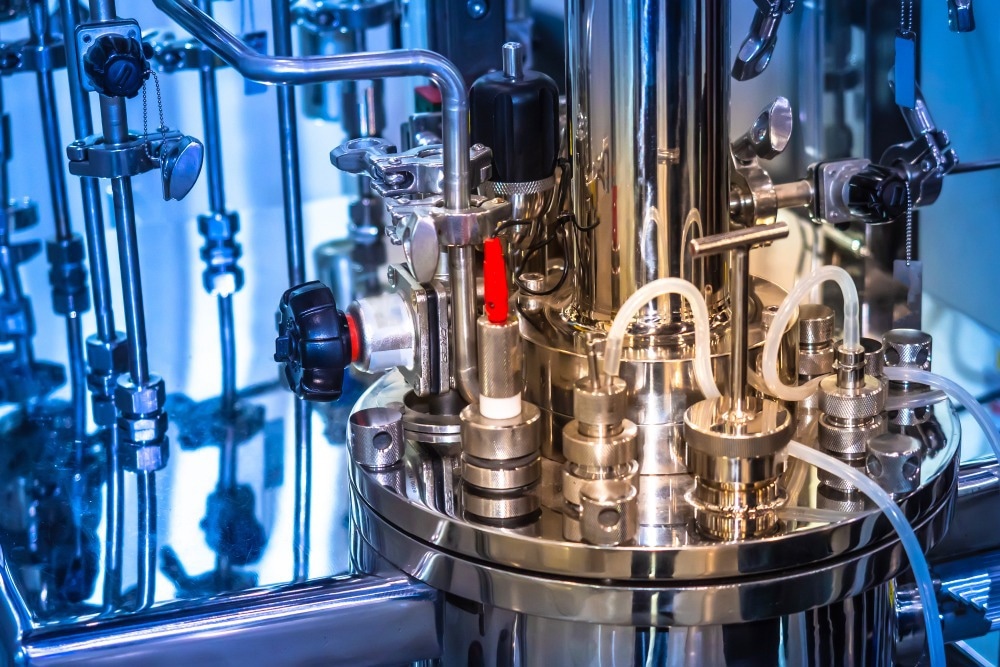
Image Credit: FOTOGRIN/Shutterstock.com
Materials science is progressing rapidly, enabling the development of novel materials, such as nanomaterials, which are employed in the fermentation industry. In addition to the novel materials, several techniques readily employed in materials science research are also used by experts in the fermentation industry to ensure the quality of fermented products.
Nanomaterials: A New Choice for Bio-hydrogen Production Using Fermentation
Over the past decade, experts have focused their attention on sustainability and using renewable materials. In this context, hydrogen produced sustainably by microorganisms, known as Biohydrogen, is finding major applications.
An article published in Bioresource Technology Reports highlights that, compared to traditional materials, modern nanomaterials used in the fermentation process result in a significant improvement in biohydrogen production.
The most commonly used methods for producing biohydrogen are photo-fermentation and photo-independent dark fermentation methods.
The photo-fermentation process involves using photosynthetic bacteria to convert organic compounds into biohydrogen and carbon dioxide.
In contrast, dark fermentation employs non-photosynthetic microorganisms to reduce protons to biohydrogen. The main challenge with dark fermentation is its low hydrogen yield.
However, iron, nickel, and their oxide nanoparticles can enhance the activity of key hydrogen production enzymes by providing raw materials for synthesis and stabilizing their molecular structure.
In a mixed bacteria fermentation system, iron and its oxide nanoparticles can enhance and select microorganisms with high hydrogen activity, indicating a preference for hydrogen-producing microorganisms by metal nanoparticles.
Iron and its oxide nanoparticles alter the physical and chemical environment of the hydrogen production system, promoting a more favorable environment for hydrogen production. In this way, experts have successfully used materials science and nanotechnology to optimize the production of biohydrogen, promoting sustainability through fermentation processes.
Material-Mediated Cell Immobilization for Biological Fermentation
Cell immobilization technology, known for its simplicity and ability to maintain long-term cell viability, repeatability, stability, and tolerance, holds significant promise in biological fermentation.
This approach involves immobilizing cells on water-insoluble carriers, supporting various life activities, including microbial growth, development, reproduction, and metabolism. It also helps mitigate the impact of external conditions on microorganisms, enabling robust metabolic activities even in challenging environments.
Natural polymer materials, such as chitosan and alginate, can be categorized into intracellular and extracellular substances. These materials, often non-toxic with favorable mass transfer and biocompatibility, are commonly employed in cell immobilization for bioproduct synthesis.
Sodium alginate, pectin, and chitosan are popular natural organic polymer carrier materials. An article published in Biofuels, Bioproducts, and Biorefining highlights how advancements in materials science are improving the fermentation process through the development of new materials and novel technologies, such as Material-Mediated Cell Immobilization.
Experts have optimized an organic material, sodium alginate, for use in bio-fermentation processes to manufacture bioethanol. The material was modified using innovative technology, resulting in significant improvements in the fermentation process. Ethanol production increased by more than 60 % compared to traditional materials and techniques.
Experts have not only optimized natural organic materials but have also produced targeted synthetic organic polymer carrier materials for microbial fermentation processes. Porous polyamide beads have been employed to immobilize Propionibacterium sp. strains for propionic acid production.
The utilization of porous polyamide beads eliminated the lag phase in cell growth, unlike fermentation with free cells, resulting in a 26.5 % increase in propionic acid production when the initial glucose concentration was 40 g/L.
The inorganic carrier material, biochar, is characterized by its rich and unique microporous structures. The biofilms generated from biochar provide a protective internal environment for microbial cells due to their intricate three-dimensional structure, thereby enhancing the cells' tolerance to adverse environmental stresses.
Recent studies have demonstrated that C. beijerinckii F-6, when immobilized on biochar, exhibits significantly higher tolerance to butanol with improved butanol production efficiency.
Compared to free-cell fermentation, the productivity of butanol and hydrogen in these studies reached 0.148 g/L/h and 0.299 mmol/L/h, respectively. This represents a 20.23 % and 48.76 % increase compared to the control. In this way, novel synthetic materials and material-mediated cell immobilization have boosted biological fermentation processes.
Chromatography Technique: From Materials Characterization to Pu-erh Tea Fermentation
Pu-erh tea is a traditional Chinese tea made via fermentation processes. The major bioactive compounds in this tea are phenolic acids, whose monitoring is crucial for maintaining quality.
An article in the Journal Of Agricultural and Food Chemistry explains that catechins, particularly EGCG, are converted into phenolic acids during the fermentation process.
To validate this claim and characterize the phenolic acids, scientists employed a new ultra-high performance DIAAA liquid chromatography technique to quantify the phenolic acids in Pu-erh tea samples during fermentation.
Notably, the retention times for DIAAA derivatives exhibited a significant increase compared to the corresponding phenolic acids. The comparison between commercial raw and ripened Pu-erh tea, along with a dynamic investigation during fermentation, revealed that simple phenolic acids generally increased while phenolic acid esters decreased post-fermentation.
Gallic acid was identified as the prominent component in ripened Pu-erh tea, whereas its ester, theogallin, was more prevalent in the raw samples. The use of liquid chromatography facilitated the efficient characterization of the phenolic acids in the tea, ensuring the quality of the final product.
How is Raman Spectroscopy Used to Monitor the Parameters of Alcoholic Fermentation?
Raman spectroscopy, long-established in materials science, has recently been used by fermentation experts to manage the quality of fermentation products, including wine. This technique is a valuable tool for ensuring the quality and safety of wine production processes.
An article in the journal Beverages highlights the application of Raman spectroscopy in monitoring the wine production process by measuring several critical parameters. The team used Raman spectroscopy to successfully predict total sugar concentrations before fermentation and post-fermentation, as well as post-fermentation ethanol and pH levels. However, it was noted that Raman spectroscopy is not particularly effective for monitoring red wine.
Raman spectroscopy generated a vast dataset, enabling the development of efficient models to predict ethanol and sugar concentration in both red and white wines. The models were created using support vector regression (SVR), partial least squares regression (PLSR), and Ridge regression (RR).
The findings demonstrated strong agreement between the anticipated and actual total sugar concentrations both before and during fermentation. The models employing SVR, PLSR, and RR exhibited high coefficients of determination (R2) and low root mean square errors (RMSE).
Similarly, when predicting ethanol and pH for finished wines after phenolic stripping with polyvinylpolypyrrolidone, the accuracy remained high, with an R2 of 0.98 for SVR and 0.99 for PLSR and RR processes.
Analyzing ethanol, pH, and total sugars in wine through Raman analysis provides a quicker alternative to other widely used analysis methods. Although the detection limit for spontaneous Raman spectroscopy is higher than the typical concentrations of common wine components, such as organic acids, this higher threshold is beneficial for creating predictive models for more abundant compounds like ethanol and total sugars. This advantage arises from the reduced risk of spectral interferences.
These findings underscore that spectroscopy is reliable for monitoring alcoholic fermentation processes.
More from AZoM: The Importance of Consistent Monitoring of Yeast Viability and Growth in Fermentation Processes
References and Further Reading
Wang, Y., et al. (2023). Application of nanomaterials in dark or light-assisted fermentation for enhanced biohydrogen production: a mini-review. Bioresource Technology Reports. doi.org/10.1016/j.biteb.2022.101295
Maicas, S. (2020). The Role of Yeasts in Fermentation Processes. Microorganisms. doi.org/10.3390/microorganisms8081142
Ge, Y., et al. (2019). Dynamic profiling of phenolic acids during Pu-erh tea fermentation using derivatization liquid chromatography–mass spectrometry approach. Journal of agricultural and food chemistry. doi.org/10.1021/acs.jafc.9b00789
Fuller H., et al. (2021). Alcoholic Fermentation Monitoring and pH Prediction in Red and White Wine by Combining Spontaneous Raman Spectroscopy and Machine Learning Algorithms. Beverages. doi.org/10.3390/beverages7040078
Gao, H., et al. (2021). Material‐mediated cell immobilization technology in the biological fermentation proces. Biofuels, Bioproducts and Biorefining. doi.org/10.1002/bbb.2219
Disclaimer: The views expressed here are those of the author expressed in their private capacity and do not necessarily represent the views of AZoM.com Limited T/A AZoNetwork the owner and operator of this website. This disclaimer forms part of the Terms and conditions of use of this website.