In this interview, Erik Schmidt-Staubach from Tinius Olsen explores the current landscape of battery and fuel cell technologies, focusing on the materials and testing required to advance these systems. They also dive into the fundamentals of fuel cells, the challenges they face, and how Intelligent Energy—an industry leader in fuel cell development—is pushing the boundaries of hydrogen technology through collaboration, research, and rigorous materials testing.
Could you start by providing a brief overview of the current battery and fuel cell technology landscape?
The race to meet net zero is becoming more important as alternatives to battery power start to come online. Materials testing is crucial for all the major components of a battery fuel cell or electrolyzer. It is a key driver in the development of improved performance and longer battery life.
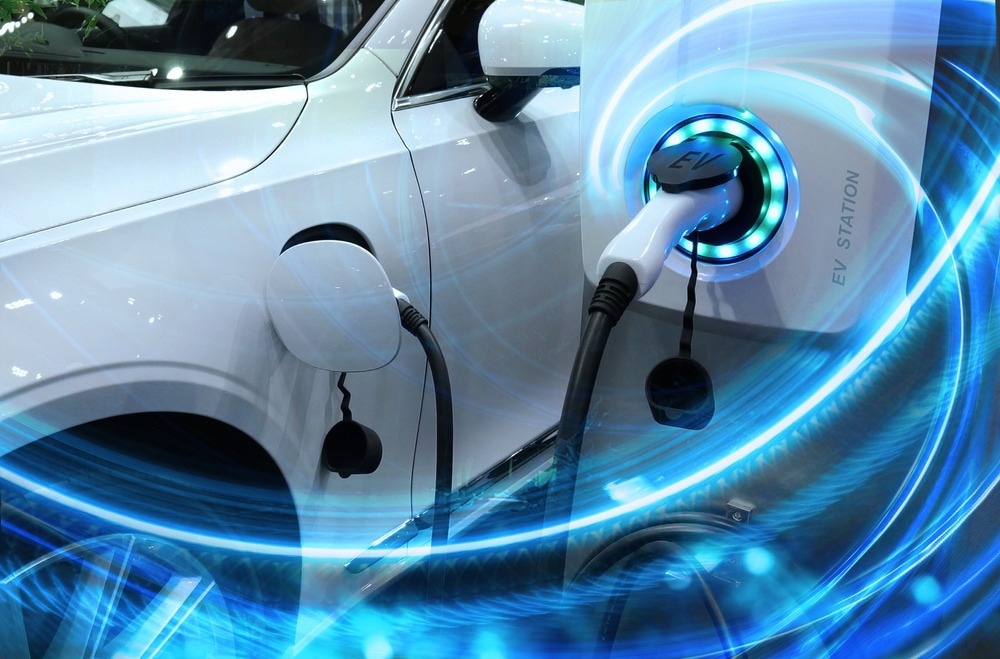
Image Credit: buffaloboy/Shutterstock.com
When former UK Prime Minister Rishi Sunak announced that “a new electric vehicle is being registered in the UK every 60 seconds,” he outlined the government’s decision to delay the ban on the sale of new petrol and diesel vehicles until 2035.
At the present rate, that is just under half a million cars a year, but the question is already being asked: which power source for the electric motor will be the best option in the drive to net zero—battery or hydrogen fuel cells?
There are several pros and cons to battery- and fuel-cell-powered vehicles. Both are extremely environmentally friendly, cheaper to run than the fossil fuel variety, require less maintenance, and some of them are extremely quick.
Batteries have some issues regarding their limited range, lifespan, cost, and the need for long charging times. Ironically, batteries can also have a negative environmental impact due to using non-sustainable electricity generation for charging and the processes and materials involved in battery production.
Electric batteries still have some issues to overcome, but fuel cell technology does not suffer from the same issues as batteries and offers more benefits; for example, refueling a fuel cell is the same as a normal weekly fill-up at the petrol station.
Neither of these technologies is new. We recognize that Welshman William Grove invented the first hydrogen fuel cell in 1838, and Alessandro Volta invented the modern electric battery 42 years earlier.
Both technologies competed in vehicular power plants until the internal combustion engine proved more convenient to use, and the die was cast for the next 140 years or so.
Fuel cell technology has a broad range of uses. For example, the aerospace industry’s aim to eventually replace fossil fuel-powered jet engines with electrically powered alternatives is becoming increasingly realistic.
How do fuel cells work, and what are their primary components?
Fuel cells work like batteries but do not run down or need recharging; they produce electricity as long as fuel is supplied. Fuel cells consist of two electrodes—a negative electrode (or anode) and a positive electrode (or cathode)—sandwiched around an electrolyte.
Fuels such as hydrogen are fed to the anode, and ambient air is fed to the cathode. In a hydrogen fuel cell, a catalyst at the anode separates hydrogen molecules into protons and electrons, which take different paths to the cathode. The electrons go through an external circuit, creating a flow of electricity, whereas the protons migrate through the electrolyte to the cathode. A catalyst at the cathode combines the protons with oxygen and the electrons to produce water vapor and heat.
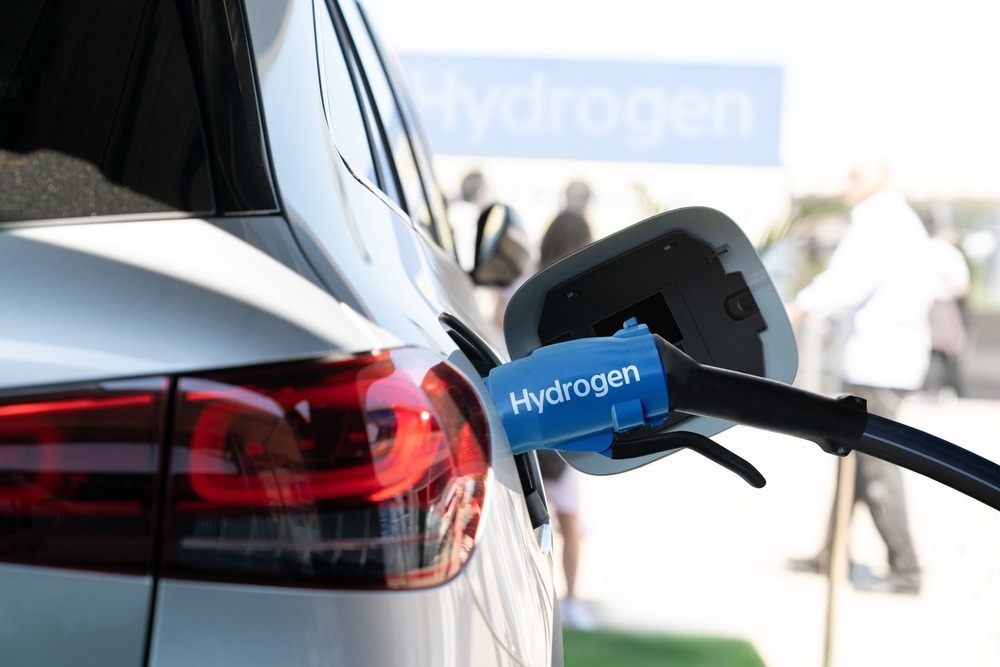
Image Credit: Scharfsinn/Shutterstock.com
It is an intrinsically simple system but highly efficient and extremely green at the tailpipe, producing only water vapor. Also, this technology does not rely on electricity from the National Grid.
More specifically, a proton exchange membrane fuel cell transforms the chemical energy liberated during the electrochemical reaction of hydrogen and oxygen into electrical energy instead of directly combusting hydrogen and oxygen gases to produce thermal energy employed by fossil fuel vehicles.
The basic components of this type of fuel cell include bipolar plates or gaskets made of metal, graphite composite, and polymer. This is a key component of the proton exchange membrane, alkaline and solid oxide fuel cells, and electrolyzers. These components are machined with complex flow fields or channels that, when stacked, distribute gas and air and conduct electrical current from one cell to the next.
Gas diffusion layers containing carbon, paper, cloth, or felt are among the most important parts of a proton exchange membrane fuel cell. They are key in transporting current to the collector plates, distributing reactant gases to the catalyst surface, and evacuating heat and water generated during the redox reactions inside the fuel cell.
A single fuel cell comprises a membrane electrode assembly and two flow-field plates delivering about 0.5 and 1 V (too low for most applications). Like batteries, individual cells are stacked to achieve a higher voltage and power. This assembly of cells is called a fuel cell stack or just a stack.
Catalyst layers (carbon/polymer) are also critical components within fuel cells, as they are the sites where the electrochemical reaction occurs.
Where does the work of Intelligent Energy sit within the wider fuel cell industry?
Intelligent Energy emerged as a spinout from Loughborough University in 2001, where the first fuel cell project began in 1988. Over the intervening years, collaborations have been formed with Suzuki, Airbus, Boeing, and, latterly, BMW in their continued development of hydrogen fuel cell applications.
Technology has advanced significantly in the last 35 years or so. Our collaborations with major global companies have contributed significantly to technological advances, and a range of market forces are continuing to drive the sector forward.
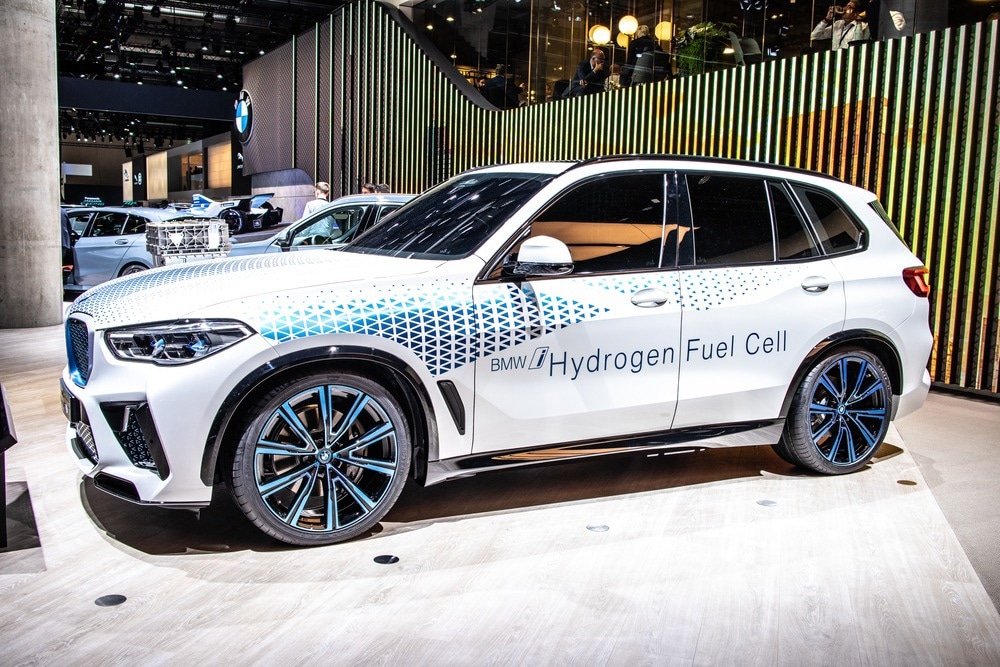
Image Credit: Grzegorz CzapskiShutterstock.com
The main considerations are reaching net zero and reducing costs. To help reach these targets, we need to produce lighter, cheaper, and even more efficient fuel cells.
This puts materials testing at the forefront of R&D because if lighter or cheaper materials work just as well as current solutions after rigorous and extensive testing, that saving can be built into the bottom line, creating a more cost-effective, viable option.
What are some of the challenges associated with historic or current hydrogen technology?
The development of hydrogen technology has faced several challenges, with hydrogen embrittlement being one of the main concerns.
Also known as hydrogen-assisted cracking or hydrogen-induced cracking, this process reduces a metal’s ductility due to absorbed hydrogen. Hydrogen atoms are small and can permeate solid metals. Once absorbed, they lower the stress required for cracks in the metal to initiate and propagate, resulting in embrittlement.
Hydrogen embrittlement occurs in steels, iron, nickel, titanium, cobalt, and alloys.
The underlying nature of hydrogen embrittlement has been documented since the 19th century. The phenomenon occurs due to the diffusion and dissolution of hydrogen in the microstructure of metals. Combined with mechanical stress, the hydrogen creates hairline cracks that grow progressively larger over time.
Steels maximize the process around room temperature, and most metals are relatively immune to hydrogen embrittlement at temperatures above 150 °C. This makes choosing materials for a given application paramount.
How can the impact of hydrogen embrittlement be analyzed and minimized?
For steels, it is important to test specimens in the lab that are at least as hard, if not harder, than the final parts. Ideally, specimens of the final material or the nearest representative should be made. Fabrication can have a profound impact on resistance to hydrogen-assisted cracking.
Stainless steels are less susceptible to hydrogen embrittlement, although a minimum nickel content of 10% is often maintained for safe use with the element. This material choice is also important if the application is subject to temperature change, such as in fuel cell technology. Testing under the right conditions, such as utilizing a heat chamber, is very important.
Numerous international standards are available for testing the effects of hydrogen embrittlement in metals and components across numerous applications, such as metal coatings, fasteners, bolts, and threads.
Two of the most used are ASTM FS19 and ISO 11114-4. The latter was originally intended as a quality standard for transporting potentially dangerous substances in gas cylinders.
The standard provides guidelines and includes methods for testing the resistance of cylinders to hydrogen embrittlement, such as Method A, also known as the disc method. This is one of the test methods specified in the standard for evaluating the susceptibility of metals to hydrogen embrittlement.
Notable failures highlight the importance of appropriate testing due to hydrogen embrittlement, such as 122 Leadenhall Street in Central London, or “The Cheesegrater” as it is commonly known. Several steel bolts used in the construction of the building were found to have suffered the effects of hydrogen embrittlement shortly after its opening in 2014, resulting in the replacement of most of the 3,000 bolts used in the construction at £6 million.
What other material testing considerations are important in fuel cell development outside of metals and the impact of hydrogen on these?
A hydrogen fuel cell uses several other materials, such as polymers, carbon, cloth, and paper. These materials require various tests, including tensile, compression, bend strength, puncture, and tear.
For example, when we develop lightweight systems for aerospace and UAV applications, we test thinner and lower-density materials to ensure they are strong and durable enough for their intended purpose.
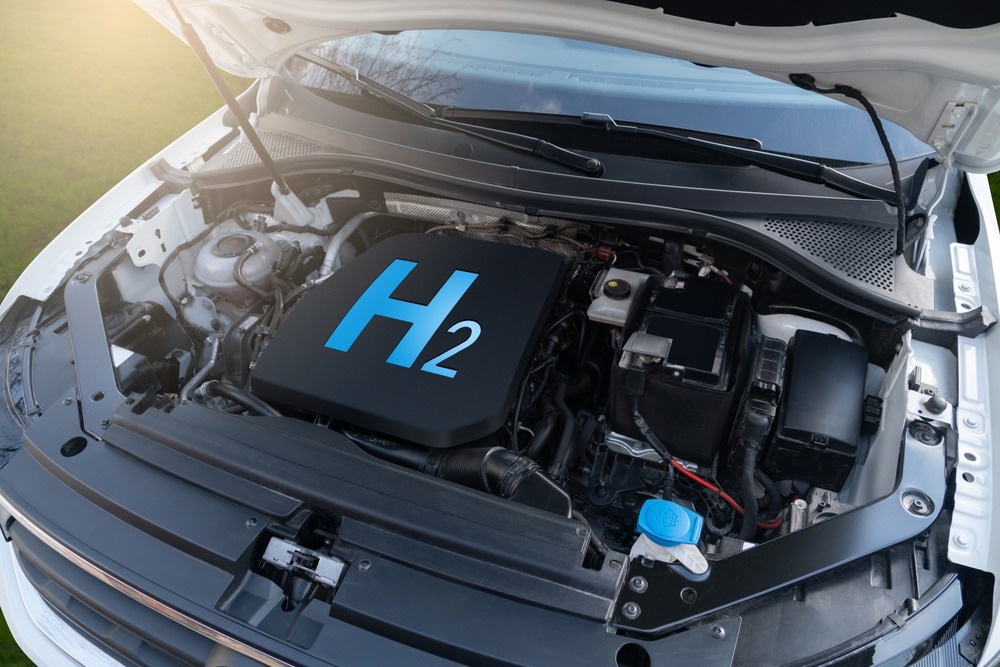
Image Credit: Scharfsinn/Shutterstock.com
This includes electrical resistance, coating testing, and the transport properties of materials such as carbon papers used for gas diffusion. For all these properties, we need to apply a range of forces to see how they will respond to changes in pressure.
Items like gaskets and seals require extensive testing because if these membranes or film materials tear or puncture during operation, the fuel cell can be irreparably damaged.
Our extensive testing program also supports research and development in areas such as mechanical design, where new designs and prototypes require extensive testing data at every stage of the process. The material properties data gleaned is then used by modeling, quality, and production teams, for example, to test product batch variability and perform defect analysis.
Our in-house research team tests the mechanical properties of materials and components, such as tensile, compressive, and bending strength or stiffness.
What are the benefits of working with a materials testing partner?
Utilizing the support of companies such as Tinius Olsen allows us greater flexibility in testing, quicker development, and more direct application. Working with industry partners has become fundamental to our work. We create and maintain a team approach to achieving results that will generate confidence in both the materials and the finished product.
Overall, Intelligent Energy and its partners have not been distracted by changes to net zero deadlines and are continuing to develop hydrogen fuel cell technology rapidly.
Major automotive manufacturers such as BMW and Toyota are actively producing their own hydrogen-powered cars, and our work will contribute to this technology by competing with, if not replacing, current battery-powered options.
About Erik Schmidt-Staubach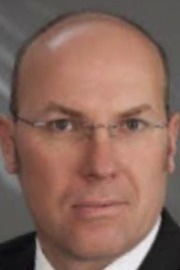
Erik is an experienced Mechanical Engineer, working within a broad range of industries including automotive, aerospace, pharmaceutical and product testing. His experience also extends to international sales, sales strategies, account management, Research & Development as well as product marketing.
About Tinius Olsen
Tinius Olsen is the leading specialist manufacturer and supplier of static tension and/or compression materials testing machines. Tinius Olsen machines are designed for use in Research and Quality Control to measure material’s strength and performance.
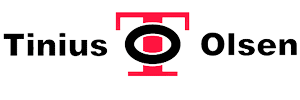
This information has been sourced, reviewed, and adapted from materials provided by Tinius Olsen.
For more information on this source, please visit Tinius Olsen.
Disclaimer: The views expressed here are those of the interviewee and do not necessarily represent the views of AZoM.com Limited (T/A) AZoNetwork, the owner and operator of this website. This disclaimer forms part of the Terms and Conditions of use of this website.