From as early as a century ago, artificial materials and devices have been developed to a point where they can be used to replace various components of the human body. These materials are capable of being in contact with bodily fluids and tissues for prolonged periods of time, whilst eliciting few, if any, adverse reactions.
Key factors in a biomaterial’s usage are its biocompatibility and functionality, which are directly related to the bone/implant interface and their nanoscale interactions. During the past two decades, improvement of these interfaces using nanoscale coatings and surface modifications have been of interest worldwide. Currently a number of companies are beginning to introduce these new-generation nanoscale modified implants into the market for orthopaedic and maxillofacial surgery, and for hard- and soft-tissue engineering.
Types of Biomaterials
When these synthetic materials are placed within the human body, the tissues react towards the implant in a variety of ways. The mechanism of tissue interaction at a nanoscale level is dependant on the response to the implant surface. As such three terms for description of a biomaterial, representing the tissues responses, have been defined. These are:
- Bioinert
- Bioresorbable
- Bioactive
The responses of these three types of biomaterials are summarised in figure 1.
![Classification of bioceramics according to their bioactivity; (a) bioinert, (alumina dental implant), (b) bioactive, hydroxyapatite (Ca10(PO4)6(OH)2) coating on a metallic dental implant, (c) surface active, bioglass or A-W glass, (d) bioresorbable tri-calcium phosphate implant [Ca3(PO4)2].](/images/Article_Images/ImageForArticle_3855_4381225742156255715.gif)
Figure 1. Classification of bioceramics according to their bioactivity; (a) bioinert, (alumina dental implant), (b) bioactive, hydroxyapatite (Ca10(PO4)6(OH)2) coating on a metallic dental implant, (c) surface active, bioglass or A-W glass, (d) bioresorbable tri-calcium phosphate implant [Ca3(PO4)2].
The Changing Role of Bioceramics
In the early 1970s, bioceramics were employed as implants to perform singular, biologically inert roles. The limitations of synthetic materials as tissue substitutes were highlighted with the increasing realisation that cells and tissues in the body perform many other vital regulatory and metabolic roles. The demands of bioceramics have since changed from maintaining an essentially physical function without eliciting a host response, to providing a more positive interaction with the host. This has been accompanied by increasing demands from medical devices to improve the quality of life, as well as extend its duration. Most importantly, nanobioceramics potentially can be used as body interactive materials, helping the body to heal, or promoting regeneration of tissues, thus restoring physiological functions.
This approach is being explored in the development of a new generation of nanobioceramics with a widened range of medical applications. Porous materials are being increasing used for bone grafts as this allows natural bone ingrowth, and a strong implant to bone bond.
Tissue Engineering Adds another Dimension
Recently, tissue engineering has taken a new direction, to take advantage of the combined use of living cells and tri-dimensional ceramic scaffolds to deliver vital cells to the damaged site of the patient. Feasible and productive strategies have been aimed at combining a relatively traditional approach, such as bioceramics implants, with the acquired knowledge applied to the field of cell growth and differentiation of osteogenic cells.
The Incorporation of Stem Cells
A stem cell is a cell from an embryo, foetus, or adult that has the ability to reproduce for long periods. It can also give rise to specialised cells that make up the tissues and organs of the body. When implanted onto immuno-deficient mice, these cells can combine with mineralised tri-dimensional scaffolds to form a highly vascularised bone tissue. Cultured-cells/bioceramic composites can be used to treat defects across the bone diaphysis with excellent integration of the ceramic scaffold with bone and good functional recovery. Excellent innovative work with nanobioceramics is in progress and clinical application is becoming quite common.
Calcium Phosphates for Bone Replacement Applications
Hydroxyapatite – The Mineral Component of Bone
The first x-ray diffraction study of bone was published by De Jong in 1926, identifying apatite as the only recognisable mineral phase. De Jong also reported marked broadening of the diffraction lines of bone apatite, which he attributed to small crystal size. Current work with a number of characterisation techniques also supports the conclusion that bone is composed essentially of carbonate substituted hydroxyapatite (CHAp).
The Composition of Inorganic Bone
The inorganic component will also contain significant but varying amounts of elements such as sodium, magnesium, and potassium. Calcium ions may occasionally also be replaced in this basic structure by atoms such as strontium. Hydroxyapatite is the idealised composition of bone, with the formula Ca10(PO4)6(OH)2. This is not a all-inclusive formula, and the apatite in bone is usually more correctly given the general formula (Ca, Mg, Na)10(PO4)3(CO3)3(OH)2.
Production of Synthetic Hydroxyapatite
Synthetic hydroxyapatite Ca10(PO4)6(OH)2 has been an attractive material for bone and tooth implants. Since its inception, one of the most common and easiest production methods of synthetic HAp has been by a solid-state reaction between Ca2+ and PO43- bearing compounds and under solution conditions in the form of powders that can be sintered to a dense polycrystalline body by firing. Parameters such as the Ca/P ratio, purity, grain size and compounds that can form during its production usually control the bioactivity of HAp. Nano- sized crystals have shown to improve bioactivity due to increased surface area.
Nanoparticulate Hydroxyapatite Materials
Some of the newly established nanotechnology companies have developed a range of nanobiomaterials incorporating nanoparticles of hydroxyapatite (HAp). The nanoparticles of HAp have opened new opportunities in designing superior biocompatible coatings for the implants and in developing high-strength nanocomposites. The nanoparticles in sizes of 15-20 nm of HAp are synthesised as ultra-pure at molecular level and have unique properties, compared to the bulk HAp.
Predicting Bioactivity of Biomaterials Using Simulated Body Fluid (SBF)
The development of a salt solution which represented similar concentrations to that of ions present in blood plasma began as early as 1943 when Earle and co-worker developed a balanced salt solution for use in cell culture media. Since then, simulated body fluids (SBF) have been produced in order to provide insight into the reactivity of the inorganic component of blood plasma, and predict the bioactivity of implants, bone scaffolds, as well as other novel biomaterials (Figure 2).

Figure 2. Schematic representation of the mechanism of apatite formation on NaOH-treated Ti metal in simulated body fluid (SBF) (From Kokubo et al., J. Aust. Ceram. Soc. 36(1), 2000, pp.37, reprinted with permission of the Australian Ceramic Society.).
Desirable Behaviour of Biomaterials in Simulated Body Fluids
Hydroxyapatite layers can be easily produced on various organic or inorganic substrates in simulated body fluid. In 1989, Kokubo and co-worker showed that after immersion in SBF, a wide range of biomaterial surfaces initiated very fine crystallites of carbonate ion-containing apatite, and since then a large number of work shows that osteoblasts can proliferate and differentiate on this apatite layer (Figure 3).
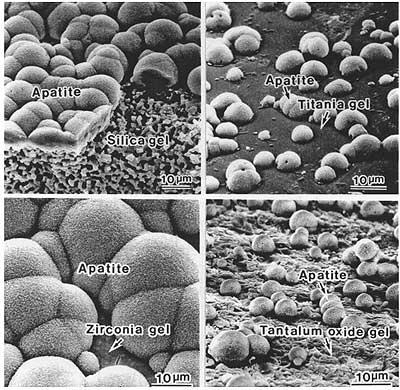
Figure 3. Apatite formation on a variety of substrates. (From kukobo et al., J. Aust. Ceram. Soc. 36(1), 2000, pp.37, reprinted with permission of the Australian Ceramic Society.).
Using Simulated Body Fluids to Deposit Apatite Layers on Biomaterials
Work has being conducted using SBF solutions to deposit apatite on both 2D and 3D scaffolds. In work by Cromme and co-worker in 2005 regenerated cellulose templates were activated with a Ca(OH)2 suspension and subsequently exposed to SBF to induce the in situ formation of biomimetic calcium phosphate phases.
Coralline Apatites
Coralline apatites can be derived from sea coral. Coral is composed of calcium carbonate in the form of aragonite. Coral is a naturally occurring structure and has optimal strength and structural characteristics. The pore structure of coralline calcium phosphate produced by certain species is similar to human cancellous bone, making it a suitable material for bone-graft applications. Coral and partially converted coralline hydroxyapatite have been used as bone grafts and orbital implants since the 1980s, as the porous nature of the structure allows in-growth of blood vessels to supply blood for bone, which eventually infiltrates the implant.
Hu and co-worker succeeded in converting Australian coral to monophasic hydroxyapatite by using a two-stage process in which the hydrothermal method was followed by a patented hydroxyapatite sol-gel coating process based on alkoxide chemistry. They reported 120% increase in the biaxial strength of this double-treated coral in comparison to the once-converted coral.
Nanocoatings
Sol-Gel Coatings
The interest in the use of sol-gel nanocoatings for implant materials is fast growing as the advantages of the sol-gel technique are numerous. It results in a stoichiometric, homogeneous and pure coating due to mixing on the molecular scale; it requires reduced firing temperatures due to nanoparticle sizes with high surface areas; it has the ability to produce uniform fine-grained structures; and the coating can be easily applied to complex shapes with a range of coating techniques, such as dip-, spin- and spray-coating.
Nanoscale Sodium Titanate Hydrogel Layers
The lower processing temperature has another advantage; it avoids the phase transition (~1156 K) observed in titanium-based alloys used for biomedical devices. Titanium metal forms a nanoscale sodium titanate hydrogel layer on its surface, when it is soaked in 5M-NaOH solution at 60°C for 24 hours. More than 70 patients have been reported to have receive artificial total hip joints of titanium alloy, modified with titanium beads subjected to NaOH treatments in Japan.
Bioceramics In In Situ Radiotherapy
One of the most common approaches in cancer treatment is the removal of the diseased parts. However, unfortunately, recovery or return of full function is seldom achieved. Non-invasive treatment techniques whereby only the cancer cells are destroyed were introduced in the mid-1980s. Microspheres of 17Y2O3-19Al2O3-64SiO2 (mol%) glass, 20-30 µm in diameter or less, were shown to be effective for in situ radiotherapy of liver cancer. 89Yttrium in this glass is non-radioactive but can be activated by neutron bombardment, to 90Y, which is a β-emitter with a half-life of 64.1 hours. The microspheres are usually injected into the diseased liver through the hepatic artery, and entrapped in small blood vessels, which block the blood supply to the cancer and directly irradiate the cancer with β -rays. Since the β -ray transmits living tissue only 2.5 mm in diameter and the glass microspheres have high chemical durability, the surrounding normal tissue is hardly damaged by the β -rays. These glass microspheres are produced and clinically used in Australia, Canada and the USA.
Biomimetics
Nature’s Way of Creating Materials
A common characteristic of natural biomaterials such as bone, nacre, sea urchin tooth and other tough hybrid materials in nature is the strong nanoscale interaction between the inorganic and the organic phases. This characteristic allows the organic phase to act as a plastic energy-dissipating network, forming stretching (bridging) ligaments across the faces of a propagating crack on a nanoscale level. Such complexity has led to the common perception that, to mimic natural designs, in situ synthesis techniques should be adopted. Precipitation of calcium carbonate or hydroxyapatite into a polymeric matrix, for example, has been proposed as a novel synthetic route to biomimetic composites.
Biomimetics and Hybrid Organic-Inorganic Biomaterials
Biomimetic or bioinspired approaches to materials and templated growth of inorganic or hybrid networks using self-assembled hybrid organic-inorganic interfaces will also help to extend the use of hybrids in the medical field. The main advantages in the use of these hybrids result from their high versatility, which offers a wide range of possibilities to elaborate tailor-made materials in terms of chemical and physical properties, and shaping. Moreover, these nanocomposites present of integrating, miniaturisation and multi-functionalisation these medical devices.
Bioengineering
At the micro/nano end of bioengineering, microtechnology and nanotechnology have been, and will be increasingly used for the fabrication of biodevices meant for emerging applications such as planar devices (e.g., array biosensors) or microfluidics devices (e.g., lab-on-a-chip). Importantly, micro/nanotechnology has been also used to fabricate advanced models for fundamental studies, such as optical manipulation of individual molecules and cells; ordered tissue engineered structures; dynamic biomolecular devices; and novel ways of computation. Many of developments in nanotechnology will result in the production of improved medical diagnostic and manipulative functional sensors.
|