Feb 26 2001
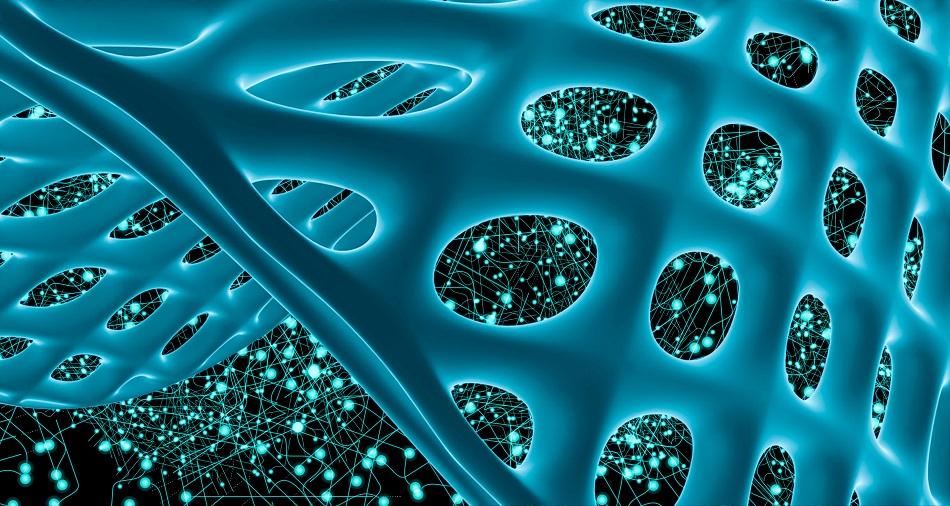
Image Credit: GiroScience/Shutterstock.com
Biomaterials improve the quality of life for the ever-increasing population. The range of applications is vast and includes joint and limb replacements, artificial arteries and skin, contact lenses, dentures, and many others. While the use of some of these materials may be for medical reasons such as the replacement of diseased tissues required to extend life expectancies, other applications are purely aesthetic – such as breast implants.
The increasing demand for these services arises from an aging population with a higher quality of life expectations. The biomaterials community is producing new improved implant materials and techniques to meet this demand, but also to aid the even more demanding treatment of younger patients. A counterforce to this technological push is the increasing level of regulation and the threat of litigation. To meet the conflicting needs, it is necessary to have reliable methods of characterization of the material and material/host tissue interactions.
Biomaterial Classifications
Biomedical materials are being roughly classified into three main types, governed by the tissue response. In broad terms, close-to-inert materials illicit no or minimal tissue response. Active materials encourage bonding to surrounding tissue through, for example, the stimulation of new bone growth. Degradable, or resorbable materials are incorporated into surrounding tissue, or maybe completely dissolved over time. Depending on the material, the tissue response of biomaterials can change: metals are typically inert; ceramics may be inert, active or resorbable; and polymers may be inert or resorbable.
Some examples of biomaterials are shown below in Table 1.
Table 1. Some accepted biomaterials
|
316L stainless steel
Co-Cr Alloys
Titanium
Ti6Al4V
|
Alumina
Zirconia
Carbon
Hydroxyapatite
|
Ultra high molecular weight polyethylene
Polyurethane
|
Key Properties
The main requirement of a biomaterial is that it does not elicit an adverse reaction when placed into service.
The range of applications for biomaterials is enormous as is the amount of different biomaterials. However, the following biomaterials are used the most:
- Metallic biomaterials are used for load-bearing applications and must have sufficient fatigue strength to endure the rigors of daily activity e.g. walking, chewing, etc.
- Ceramic biomaterials are appreciated for their hardness and wear resistance for applications such as articulating surfaces in joints and teeth, as well as bone-bonding surfaces in implants.
- Polymeric materials are usually used for their flexibility and stability but have also been used for low friction articulating surfaces.
Applications
The following sections provide some details on biomaterials’ applications. For even more details and greater depth on the topic, refer to particular materials or applications.
Orthopedic Applications
Metallic, ceramic, and polymeric biomaterials are often implemented in the field of orthopedics. Metallic materials are normally used for load-bearing members such as pins, plates, femoral stems, etc. Ceramics such as alumina and zirconia are used for wear applications in joint replacements, while bone-bonding applications that assist implant integration normally contain hydroxyapatite. Polymers such as ultra-high molecular weight polyethylene are used as articulating surfaces against ceramic components in joint replacements.
Porous alumina also has an application as a bone spacer to replace large sections of bone which are removed as a result of a disease.
Dental Applications
Metallic biomaterials have been used as pins for anchoring tooth implants and as parts of orthodontic devices. Ceramics have found uses as tooth implants including alumina and dental porcelains. Hydroxyapatite has been used for coatings on metallic pins and to fill large bone voids from disease or trauma. Polymers are also orthodontic devices such as plates and dentures
Cardiovascular Applications
Many different biomaterials play a role in cardiovascular applications depending on the specific application and the design. For instance, the carbon in heart valves and polyurethanes for pacemaker leads serve as insulators. However, research shows that polyurethane cardiovascular implants have the tendency and disadvantage of oxidizing, and therefore degrading, in vivo, creating serious problems after the implantation.
Cosmetic Surgery
Materials like silicones have been widely utilized in cosmetic surgery for applications such as breast augmentation.
Sources and Further Reading
This article was updated on 29th January, 2020.