The latest generation of field emission scanning electron microscopes (FE-SEM) have now taken a significant evolutionary step towards the improvement of resolution possibilities. High resolution combined with an additional expansion of analytical capabilities is extremely important for many applications in material analysis. With the integration of a charge compensation system these investigations are not restricted to conductive samples only but can also be executed for all kinds of nonconductive samples.
Field Emission Scanning Electron Microscopes from Carl Zeiss
Carl Zeiss provides a wide range of FE-SEM systems designed to meet a wide diversity of customer demands. For maximum versatility, the ULTRA PLUS and MERLIN® FE-SEM, as well as the AURIGA® CrossBeam® system are equipped with a pneumatically insertable gas injection system [Fig. 1, 2]. A bendable stainless steel needle locally injects gas into the region of interest (ROI). The spatial limitation of the low vacuum area allows the use of all detectors that are normally used in high vacuum conditions. Furthermore skirt effect issues, e.g. significant deterioration of image quality and reduction of resolution by beam broadening due to scattering, are drastically reduced in comparison to environmental systems where the chamber is at low vacuum entirely and, hence, the interaction volume is much larger. The needle can be adjusted in all directions. With its bayonet coupling it can easily be exchanged for alternative shapes that can be used for special applications such as EBSD.
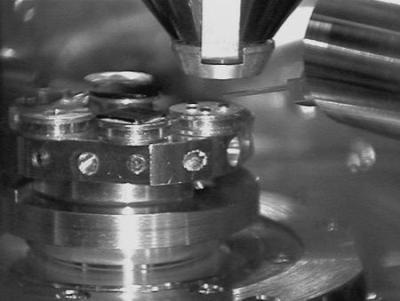
Fig. 1. TV image of the charge compensation system inserted into the chamber.
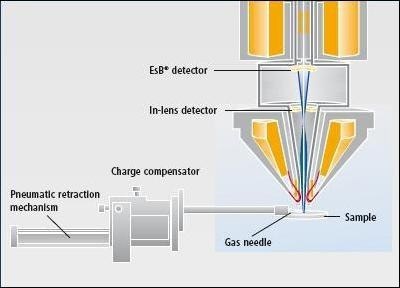
Fig. 2. Fast change between local charge compensation and high vacuum operation is guaranteed by a simple pneumatic retraction mechanism for the gas injection system. All detectors can be used under both conditions.
Basic Principle of Charge Compensation
Charging is the crucial issue when it comes to investigating non-conductive specimens. Depending on the primary electron energy an insulator, when not in charge equilibrium, can charge up positively (at lower energies than the potential at equilibrium of charge) or negatively (at higher energies). To charge up positively (or negatively) the total electron yield, i.e. the number of electrons leaving the specimen, is greater (or less) than the number of primary electrons hitting the specimen. As a result, a positively charged area appears dark as the emission of secondary electrons is suppressed. Conversely, a negatively charged area appears bright. Normally, the situation is even more complicated because charging is a dynamic rather than a static process.
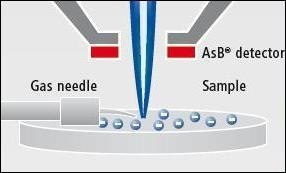
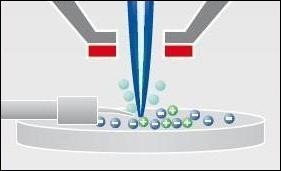
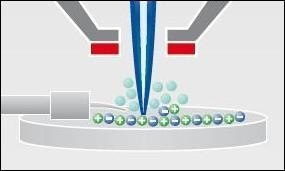
Fig. 3. Principle of charge compensation: Top: The sample surface of an insulator charges up due to electron irradiation. Middle: When the nitrogen flow is turned on, the gas molecules (light green) form a local gas cloud above the sample surface. Bottom: SE and BSE emitted from the sample surface ionize the gas molecules. As the resulting positive ions (dark green) hit the sample surface it is neutralized. Full imaging and analytical capabilities are thus enabled.
Deposition of a thin conductive layer by evaporation or sputtering was historically used to overcome charging issues. But more time has to be spent for sample preparation while surface details are covered with the additional layer, also influencing material analytics. Surface sensitive methods such as EBSD become impossible. Environmental or variable pressure systems can be used instead, where the whole chamber is flooded with gas (mostly nitrogen or water vapor) that is ionized by secondary electrons (SE) and low-energy backscattered electrons (BSE) close to the sample surface [Fig. 3]. Due to the comparably small ionization cross-section of the primary beam high energy electrons will not significantly contribute to the process [2]. Positively charged ions and negatively charged electrons then recombine on the sample surface leading to charge neutralization. A disadvantage of this method is the large interaction volume. Scattering leads to beam broadening and, hence, worse resolution. Additionally, the number of detectors that can be used under low vacuum conditions is limited to semiconductors and dedicated low vacuum detectors only. Scintillators – the most common type of detectors – can not be used as their activation potential of approx. 10 kV at the crystal layer would lead to arching.
Charge Compensation System from Carl Zeiss
With the Carl Zeiss charge compensation system gaseous nitrogen is injected into the vicinity of the ROI, limiting the area of lower vacuum to a very small volume. While the mechanism to avoid charging is the same, the vacuum in the chamber is still good enough, so that all high vacuum detectors can still be used. Image acquisition can be done without encountering skirt effect issues, e.g. significant deterioration of image quality and reduction of resolution [Fig. 4]. Investigations are even possible at high magnifications. As the dose of irradiation increases the gas pressure has to be increased accordingly [Fig. 5].
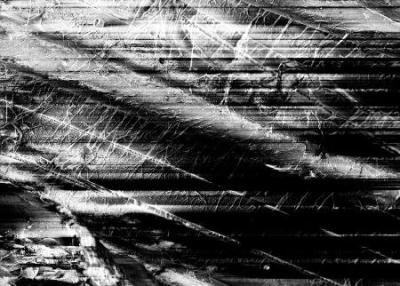
Fig. 4a. Uncoated, non-conductive glass fibers with epoxy imaged without using charge compensation. Chamber SE detector, 2 kV, WD 6 mm: strong image distortion.
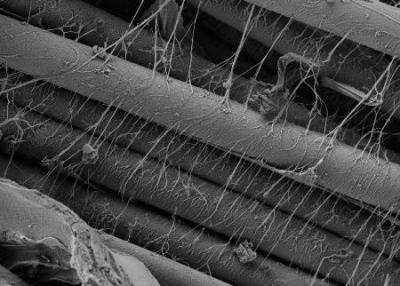
Fig. 4b. Uncoated, non-conductive glass fibers with epoxy imaged while using charge compensation. Chamber SE detector, 2 kV, WD 6 mm: flawless image quality.
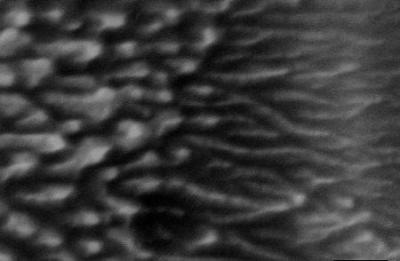
Fig. 5. Detail of paper fiber, In-lens detector, 4 kV, WD 4 mm: charge compensation is possible even at high magnifications, if scan mode, current and gas pressure are chosen carefully.
Additionally, charging does not only reduce image quality and surface information but also influences analytical results. A negative potential on the surface acts as a retarding field reducing the energy of the primary electrons. This shielding effect shifts the cut-off voltage of bremsstrahlung (Duane-Hunt-Limit) to lower energies, which has a significant influence on element analysis by EDS. With the charge compensation system any effect of charging on analytical results can be prevented [Fig. 6].
Experiments show that the introduction of the steel needle into the electrostatic field of the lens has more influence on resolution than the gas flow [Fig. 7]. But even at low energies of 1 keV, resolution is not decreased by more than 0.7 nm (at 100% gas flow). For average operating conditions energies of more than 2 keV and gas flows below 100% are used. A deteriorating effect of charge compensation is kept to a minimum.
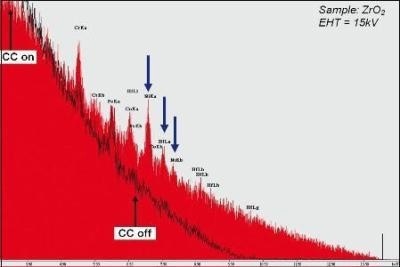
Fig: 6. Effects of charging on an EDX spectrum of non-conductive ZrO2: The Duane-Hunt limit is shifted down to lower voltages. Several emission lines show reduced intensity while some are not visible at all. When using the charge compensation the Duane-Hunt limit is not affected at all, all information becomes accessible.
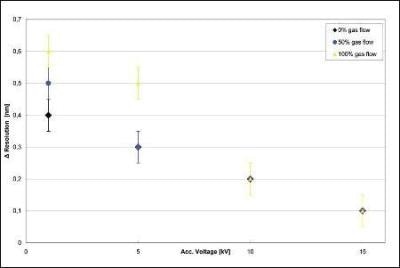
Fig. 7. Change of resolution values due to the use of the charge compensation system (working distance: 3.5 mm): even at extreme operating conditions deterioration is minimal. The needle is the main influencing factor, rather than the flow of nitrogen.
Other Applications of Charge Compensation System
The Carl Zeiss gas injection system can be used equally well with oxygen instead of nitrogen. This offers a unique opportunity for in-situ reduction of contamination, making investigations even more time-effective and efficient avoiding prolonged preparation time of specimen contaminated prior to loading (see application note “In-situ Cleaning“).
Summary
The Carl Zeiss charge compensation system offers localized decharging of non-conductive specimens by ionization of nitrogen. All high vacuum detectors can still be used for investigations, making a comprehensive analysis of specimens time-saving and easy even for novice users.
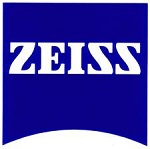
This information has been sourced, reviewed and adapted from materials provided by Carl Zeiss Microscopy GmbH.
For more information on this source, please visit Carl Zeiss Microscopy GmbH.