The subject of hybrids based on layered inorganic compounds such as clays has been studied for a considerable time, but the area is enjoying a resurgence of interest and activity as a result of the exceptional properties which can be realised from such nanocomposites. Materials variables which can be controlled and which can have a profound influence on the nature and properties of the final nanocomposite include the type of clay, the choice of clay pre-treatment, the selection of polymer component and the way in which the polymer is incorporated into the nanocomposite. The last of these may be dictated by the available processing methods and whether the prospective user is an integrated polymer manufacturer or a specialist processor. Clays and Clay Modifications Common clays are naturally occurring minerals and are thus subject to natural variability in their constitution. The purity of the clay can affect final nanocomposite properties. Many clays are aluminosilicates, which have a sheet-like (layered) structure, and consist of silica SiO4 tetrahedra bonded to alumina AlO6 octahedra in a variety of ways. A 2:1 ratio of the tetrahedra to the octahedra results in smectite clays, the most common of which is montmorillonite. Other metals such as magnesium may replace the aluminium in the crystal structure. Depending on the precise chemical composition of the clay, the sheets bear a charge on the surface and edges, this charge being balanced by counter-ions, which reside in part in the inter-layer spacing of the clay. The thickness of the layers (platelets) is of the order of 1 nm and aspect ratios are high, typically 100-1500. The clay platelets are truly nanoparticulate. In the context of nanocomposites, it is important to note that the molecular weight of the platelets (ca. 1.3 x 108) is considerably greater than that of typical commercial polymers, a feature which is often misrepresented in schematic diagrams of clay-based nanocomposites. In addition, platelets are not totally rigid, but have a degree of flexibility. The clays often have very high surface areas, up to hundreds of m2 per gram. The clays are also characterised by their ion (e.g. cation) exchange capacities, which can vary widely. One important consequence of the charged nature of the clays is that they are generally highly hydrophilic species and therefore naturally incompatible with a wide range of polymer types. A necessary prerequisite for successful formation of polymer-clay nanocomposites is therefore alteration of the clay polarity to make the clay ‘organophilic’. An organophilic clay can be produced from a normally hydrophilic clay by ion exchange with an organic cation such as an alkylammonium ion. For example, in montmorillonite, the sodium ions in the clay can be exchanged for an amino acid such as 12-aminododecanoic acid (ADA): Na+-CLAY + HO2C-R-NH3+Cl- →.HO2C-R-NH3+-CLAY + NaCl The way in which this is done has a major effect on the formation of particular nanocomposite product forms and this is discussed further below. Although the organic pre-treatment adds to the cost of the clay, the clays are nonetheless relatively cheap feedstocks with minimal limitation on supply. Montmorillonite is the most common type of clay used for nanocomposite formation; however, other types of clay can also be used depending on the precise properties required from the product. These clays include hectorites (magnesiosilicates), which contain very small platelets, and synthetic clays (e.g. hydrotalcite), which can be produced in a very pure form and can carry a positive charge on the platelets, in contrast to the negative charge found in montmorillonites. Synthetic Processing of Clay-Based Nanocomposites The synthetic route of choice for making a nanocomposite depends on whether the final material is required in the form of an intercalated or exfoliated hybrid (Figure 1). In the case of an intercalate, the organic component is inserted between the layers of the clay such that the inter-layer spacing is expanded, but the layers still bear a well-defined spatial relationship to each other. In an exfoliated structure, the layers of the clay have been completely separated and the individual layers are distributed throughout the organic matrix. A third alternative is dispersion of complete clay particles (tactoids) within the polymer matrix, but this simply represents use of the clay as a conventional filler. 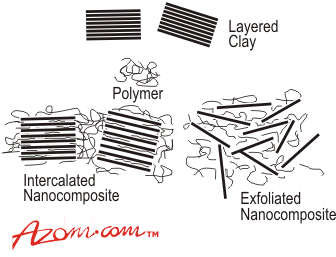 | Figure 1. Formation of intercalated and exfoliated nanocomposites from layered silicates and polymers (not to scale). | Factors Affecting the Type of Organo-Clay Hybrid Formed In recent years, there has been extensive study of the factors which control whether a particular organo-clay hybrid can be synthesised as an intercalated or exfoliated structure. Since clay nanocomposites can produce dramatic improvements in a variety of properties, it is important to understand the factors which affect delamination of the clay. These factors include the exchange capacity of the clay, the polarity of the reaction medium and the chemical nature of the interlayer cations (e.g. onium ions). By modifying the surface polarity of the clay, onium ions allow thermodynamically favourable penetration of polymer precursors into the interlayer region. The ability of the onium ion to assist in delamination of the clay depends on its chemical nature such as its polarity. The loading of the onium ion on the clay is also crucial for success and it should be borne in mind that a commercial organo-clay may not have the optimum loading for a particular application. For positively charged clays such as hydrotalcite, the onium salt modification is replaced by use of a cheaper anionic surfactant. Other types of clay modification can be used depending on the choice of polymer, including ion-dipole interactions, use of silane coupling agents and use of block copolymers. An example of ion-dipole interactions is the intercalation of a small molecule such as dodecylpyrrolidone into the clay. Entropically-driven displacement of the small molecules then provides a route to introducing polymer molecules. Unfavourable interactions of clay edges with polymers can be overcome by use of silane coupling agents to modify the edges. These can be used in conjunction with the onium ion treated organo-clay. An alternative approach to compatibilising clays with polymers has been introduced by TNO (Netherlands), based on use of block or graft copolymers where one component of the copolymer is compatible with the clay and the other with the polymer matrix. This is similar in concept to compatibilisation of polymer blends. A typical block copolymer would consist of a clay-compatible hydrophilic block and a polymer-compatible hydrophobic block (figure 2). 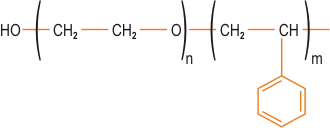 | Figure 2. Structure of a typical polymer-compatible hydrophobic block. | The block length must be controlled and must not be too long. High degrees of exfoliation are claimed using this approach. Polymer Incorporation The correct selection of modified clay is essential to ensure effective penetration of the polymer or its precursor into the interlayer spacing of the clay and result in the desired exfoliated or intercalated product. Indeed, further development of compatibiliser chemistry is undoubtedly the key to expansion of this nanocomposite technology beyond the systems where success has been achieved to date. Polymer can be incorporated either as the polymeric species itself or via the monomer, which is polymerised in situ to give the corresponding polymer-clay nanocomposite. The second of these is the most successful approach to date, although it probably limits the ultimate applicability of these systems. Polymers can be introduced either by melt blending, for example extrusion, or by solution blending. Melt blending (compounding) depends on shear to help delaminate the clay and can be less effective than in situ polymerisation in producing an exfoliated nanocomposite. Both thermosets and thermoplastics have been incorporated into nanocomposites, including: • Nylons • Polyolefins, e.g. polypropylene • Polystyrene • Ethylene-vinyl acetate (EVA) copolymer • Epoxy resins • Polyurethanes • Polyimides • Poly(ethylene terephthalate) (PET) Clay-Nylon Nanocomposites The earliest example of the in situ polymerisation method was work by Toyota on synthesis of clay-nylon nanocomposites and this remains probably the most studied system, including work by Bayer and Ube. In a typical synthesis ADA-modified clay is dispersed in the monomer caprolactam, which is polymerised to form the nylon-6-clay hybrid as an exfoliated composite. Complete exfoliation may be preceded by intercalation of the monomer in the clay. Generally, low concentration of clay (a few %) are incorporated in these nanocomposites, partly because this is often sufficient to modify the desired properties significantly, but also because higher levels of clay can adversely increase the system viscosity leading to poor processability, although the viscosity increase is shear rate dependent. Other nylons and copolyamides (e.g. nylon-6/6,6) have also been incorporated in clay nanocomposites. Functionality such as hydroxyl groups can be introduced into the onium salt modifiers to improve compatibility with the nylon via hydrogen bonding – this can lead to an enhancement of desirable nanocomposite properties. Similar modification of the chemistry may be required for successful exfoliation with other types of polymer system. In the case of ethylene-vinyl alcohol (EVOH) copolymers, use of hydroxylated quaternary ammonium ions improves compatibility between the clay and the EVOH by introducing favourable hydroxyl group interactions. In polypropylene (PP) nanocomposites, maleic anhydride grafted PP is used as a compatibiliser. Polymerisation initiators can be anchored to the clay platelet surface and this approach has been extended to living free radical polymerisation of styrene, where an initiating species bonded to the TEMPO free radical is attached to the surface of the clay platelets via ion exchange. High Temperature Thermoplastics in Clay-Based Nanocomposites For preparation of nanocomposites from high temperature engineering thermoplastics, a major limitation in the use of conventional onium ion modified clays is the thermal instability of the alkylammonium species during processing. The block copolymer route developed by TNO (vide supra) offers one potential solution to this problem. Imidazolium salts such as that depicted in Figure 3 are also more thermally stable than the ammonium salts. 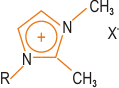 | Figure 3. Structure of an imidazolium salts. | A further alternative is the use of phosphonium salts in place of ammonium salts – this can lead to an increase in the degradation temperature of the organo-clay from 200-300°C to >300°C. Wholly synthetic organo-clays clays, on the other hand, can exhibit thermal stability to beyond 400°C. By using these last two approaches, Triton Systems has succeeded in producing nanocomposites from high temperature resins such as polyetherimide (PEI). Forming the nanocomposite provides a potential route to producing ‘speciality’ engineering resin performance from cheaper ‘standard’ engineering resins. The Future Future goals include the extension of this nanotechnology to additional types of polymer system, where the development of new compatibilisation strategies is likely to be a prerequisite. Production of PVC-based systems is still some way off and challenges remain to be solved in PET nanocomposites. Additional reinforcement of clay nanocomposites by glass fibre is currently being investigated. There is also interest in the development of electrically conducting clay nanocomposites. |