The RF focusing fields in the Vocus ion molecule reactor improve sensitivity while maintaining product ion energies and recorded branching ratios that are nearly identical to those of traditional drift-tube PTR ion-molecule reactor designs.
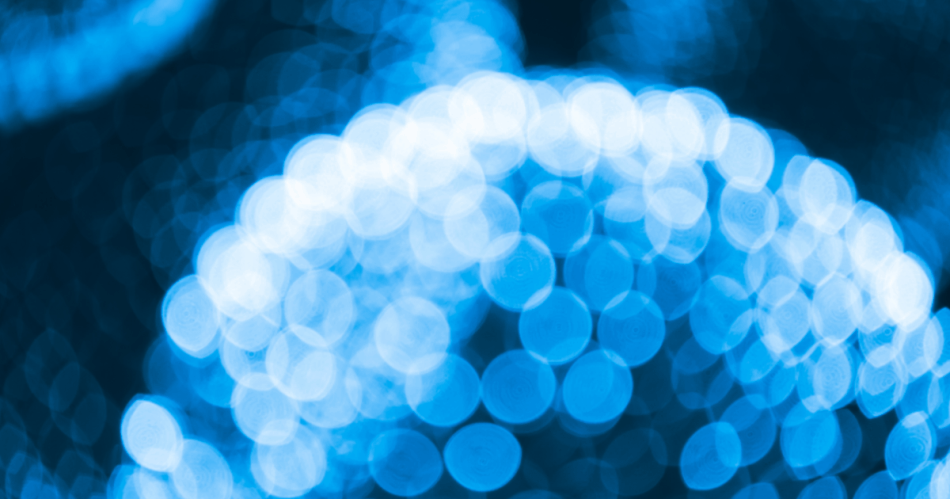
Felipe Lopez-Hilfiker, Liang Zhu, Manuel Hutterli, Carsten Stoermer,
Luca Cappellin
TOFWERK, Thun, Switzerland
Increases in the sensitivity of proton transfer reaction mass spectrometers (PTR-MS) have stemmed mainly from upgrades of mass analyzers and improvements in the ion transfer stages that couple the PTR ion-molecule reactor (IMR) to the mass analyzer. Only recently has attention shifted to the design of the PTR IMR, which was largely unchanged over the past decade.
Traditional PTR IMRs – often called drift-tube IMRs – consist of stacked ring electrodes connected by a resistor network to generate a linear electrostatic field. In combination with the precisely controlled IMR pressure and temperature, this electric field defines ion-molecule collision energies, reactant ion distributions and reaction times. The collision conditions in drift tube IMRs can be calculated from first principles, making it straightforward to predict the energy and fragmentation of product ions. But, >90% of product ions are lost in the IMR due to diffusion and scattering, significantly limiting instrument sensitivity.
The TOFWERK Vocus ion molecule reactor1 was developed to overcome these types of ion losses. Oscillating radio frequency (RF) fields are superimposed on the linear drift field to increase ion transmission through the IMR and thereby improve PTR-MS sensitivity. In any ion guiding device that uses RF fields, the instantaneous electric field experienced by an ion depends on its mass and the distance to the RF electrode. In contrast to a drift-tube IMR, this added complexity makes it difficult to calculate the net collision conditions, leading to potential errors in quantification and high fragmentation ratios if improperly designed or used. This note reviews the design of the Vocus IMR, discusses the electric fields experienced by ions in the IMR, and compares collision energies and fragmentation patterns under standard operating conditions to those of drift tube IMRs.
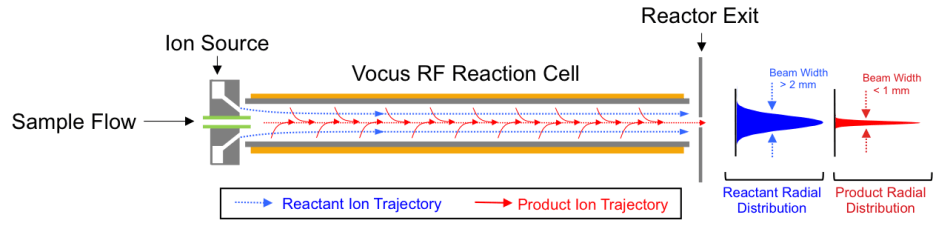
Figure 1 Schematic of Vocus IMR showing ion trajectories for both reactant ions and product ions (greater than 50 Th). The resistive-glass reaction cell (grey) is housed inside the four rods of an rf-only quadrupole (orange). Low mass-to-charge reactant ions (19 Th, blue) reactant ions are not as tightly focused as higher mass-to-charge product ions (red), which are rapidly focused to the central axis by the applied RF field.
The Vocus Reactor Superimposes RF Focusing Fields on Axial Drift Fields
The TOFWERK Vocus ion molecule reactor, shown schematically in Figure 1, consists of a resistive-glass tube reaction cell (grey) housed inside a quadrupole ion guide (yellow). Upstream of the IMR, water vapor is passed through a low-pressure plasma to generate H3O+ reactant ions in high abundance. These ions enter the IMR via a quenching region which ensures high reactant ion purity.2 Sampled air enters the IMR through a capillary tube located on the reactor axis. Transfer of protons from the H3O+ reactant ions to neutral analyte molecules forms product ions during the ~100 µs transit through the cell. The traditional axial electrostatic field is applied across the length of the glass tube to control reaction time and collision conditions. The superposition of an RF field with this axial DC field focuses product ions into a narrow beam for efficient transfer through the exit orifice.
Product Ions in the Vocus IMR are Rapidly Focused and Undergo Limited Heating
The RF field strength experienced by ions in the Vocus IMR depends on their radial positions relative to the central axis of the glass reaction cell. The larger the distance of an ion from center, the more energy it gains from the RF. Such heating of unfocused ions could potentially drive fragmentation or complicate the ion chemistry. As depicted in Figure 1, though, the quadrupole field used in the Vocus IMR rapidly focuses product ions close to the central axis where the net RF field strength is 0V.
This is demonstrated in Figure 2, which shows the radial distribution of product ions with mass/charge of 100 Th in the Vocus reactor operated at 2 mbar and the corresponding axial and radial kinetic energies, as modeled by SIMION. The axial energy (red) is driven by the DC electric field between the two ends of the IMR and has little dependence on radial position. The radial energy (black), which is driven by the RF field, is zero at the center axis and rises rapidly as ions move away from center. Focusing by the RF fields produces a tight spatial distribution of product ions (blue), with most ions confined near the center axis, such that the energy gained due to the RF is limited and therefore collision conditions are largely unchanged compared to conventional drift-tube PTR reactors.
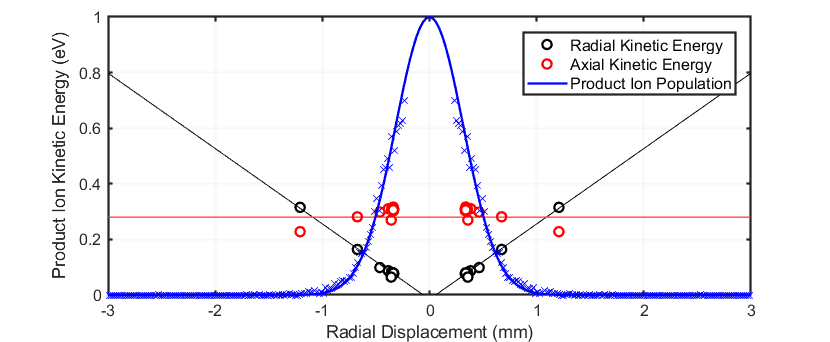
Figure 2 (blue) Radial distribution of product ions with mass/charge of 100 Th in a Vocus reactor operated at 2 mbar and (black) radial and (red) axial components of ions’ kinetic energies as modeled by SIMION. The radial component of the energy, which is driven by the RF field, is zero at the central axis and increases with radial displacement. The axial kinetic energy is driven by the DC field that is applied along the reactor axis and has little dependence on radial position. Product ions are rapidly focused to the central axis by the RF field, so that net heating due to the applied RF is very limited and overall kinetic energy is similar to what is experienced with only a DC drift field.
RF Focusing Improves Sensitivity without Increasing Fragmentation
α-Pinene (C10H16) has been frequently measured by PTR-MS using traditional drift tube ion molecule reactors3 and is known to have one major fragment product ion (C6H9+). The relatively intensities of this fragment and the molecular ion (C10H16H+) depend strongly on the net collision conditions in the reaction cell. Measurement of these intensities can be used to characterize the net heating in the Vocus IMR and to compare the Vocus to traditional systems.
Figure 3 shows the effect of scanning the Vocus RF amplitude (at fixed frequency) on the α-Pinene branching ratio and observed sensitivity at a typical and an elevated IMR operating pressure. The upper-left panel shows recorded branching ratios at 2 mbar with comparison to literature values for a drift tube IMR3 (shown as solid dots at amplitude = 0V). As modeled in Figure 2, the RF field rapidly focuses ions to the central axis at this pressure. Because radial heating is negligible near the center, the effective ion temperature does not change as a function of RF amplitude, and the branching ratio remains close to the published drift-tube value even at high amplitudes. At the same time, the increase in RF improves focusing and transmission out of the IMR, yielding a > 10 x improvement in sensitivity compared to operations with only an axial drift field.
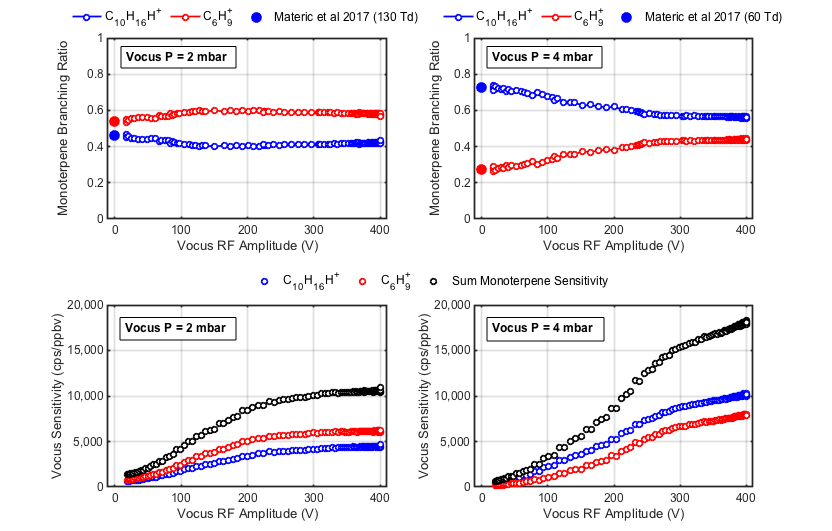
Figure 3 The effect of the applied RF amplitude in a Vocus IMR on observed (top) fragmentation and (bottom) sensitivity at (left) standard and (right) elevated operating pressures. Filled points in the upper plots show published values of the branching ratios of a traditional drift tube at these pressures. At low pressures, the RF efficiently focuses the product ion beam along the central axis where radial heating is near zero, and product ions are transferred through the reactor in the “drift tube limit” even at high RF. At higher pressure, data show less overall fragmentation because ion-molecule collisions are lower in energy. The RF field is less efficient at focusing product ions to the central axis, and fragmentation increases slightly with increased RF. Absolute sensitivity increases by more than an order of magnitude with applied RF at both pressures, improving measurement quality and speed.
The panels on the right display the same data recorded at a Vocus IMR pressure of 4 mbar. Overall sensitivity increases with the increase in pressure because ion-neutral collisions become more frequent. Collisions also become less energetic, leading to less fragmentation compared to the lower pressure operation. With no applied RF, the spectrum is dominated by the molecular ion and consistent with the published value. Focusing by the RF field is less effective at this pressure and product ions are – on average – farther from the central axis than in the 2-mbar case. Because more ions are in the region where radial heating is significant, the increase in RF does increase the observed degree of fragmentation (although the molecular ion does remain dominant even at high RF). Similar to the 2 mbar experiment, the addition of the RF fields leads to a substantial improvement in sensitivity.
Conclusions
The Vocus ion molecule reactor uniquely uses RF focusing fields to overcome diffusional ion losses that are typical of conventional drift-tube reactors used in PTR-MS. This focusing increases sensitivity more than 10-fold. At typical operating pressure (~2 mbar), product ions are rapidly focused along the central axis of the Vocus reactor, where ions do not experience additional heating from the applied RF fields. In this regime, product ion energies and recorded branching ratios are nearly identical to those recorded with traditional drift-tube ion molecule reactor designs.
References
- Krechmer, J.; Lopez-Hilfiker, F.; et al., Evaluation of a new Vocus reagent-ion source and focusing ion-molecule reactor for use in proton-transfer-reaction mass spectrometry. Anal. Chem., 2018. 90 (20), 12011. 10.1021/acs.analchem.8b02641
- High purity H3O+ reactant ions enable simple interpretation of Vocus PTR-TOF data., White Paper, TOFWERK, 2019, https://www.tofwerk.com/vocus-high-purity-reactant-ions/
- Materić D; et al., Monoterpene separation by coupling proton transfer reaction time-of-flight mass spectrometry with fastGC. Anal. Bioanal. Chem., 2015, 407 (25), 7757. 10.1007/s00216-015-8942-5