By Surbhi JainReviewed by Susha Cheriyedath, M.Sc.Apr 22 2022
In an article recently published in the Journal of Energy Chemistry, researchers discussed the gas evolution and relationship with electrode materials in a rapid operando gas monitor for commercial real-size lithium-ion batteries.
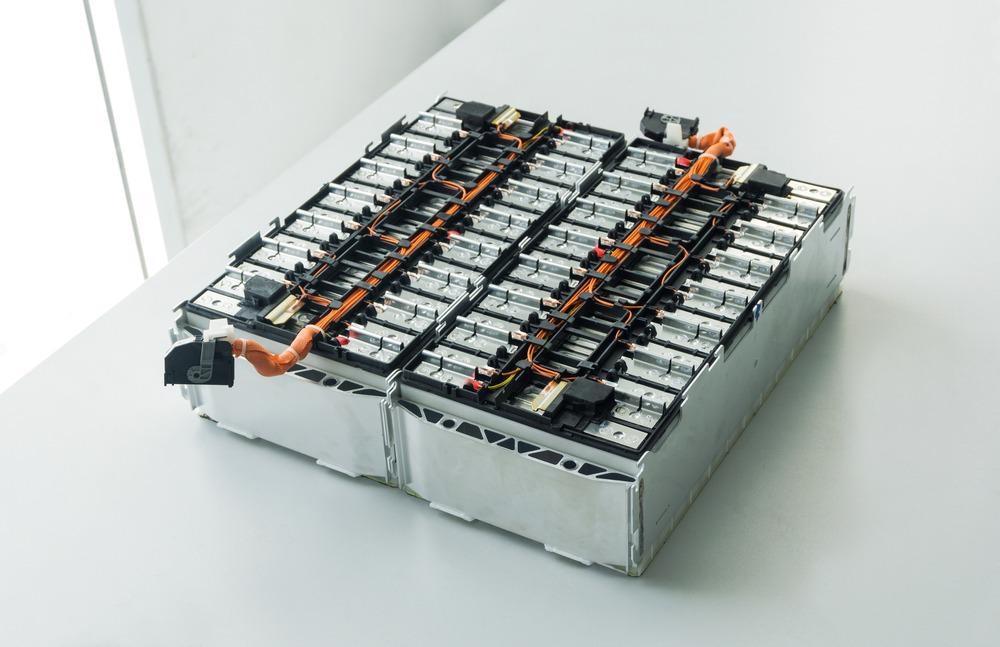
Study: Rapid operando gas monitor for commercial real-size lithium ion batteries: Gas evolution and relation with electrode materials. Image Credit: Smile Fight/Shutterstock.com
Background
Because of their good cycling ability, high energy density, eco-friendliness, high operating voltage, and low self-discharge, Li-ion batteries (LIBs) are known to be important energy storage technologies and have been extensively used in electric vehicles, portable electronics, and energy storage systems.
Positive electrode materials based on LiNixMnyCo1xyO2 (NMC) are suitable for obtaining high-energy-density LIBs; however, they are prone to degradation due to structural and chemical instability. There have been numerous comprehensive and extensive examinations of gas evolution mechanisms published.
The majority of gases are studied during the battery's construction, first cycle, and thermal runaway phase, which is based on harsh conditions such as high temperatures above 90 °C and/or overcharging. However, the evolution of gases inside the real-size LIBs under normal operating settings has not been thoroughly described, despite the fact that understanding the early stages prior to battery failure or thermal runaway is critical.
About the Study
In this study, the authors discussed the development of a novel method for fast operando monitoring gas evolution to address the challenges of implanting various gas sensors into commercial batteries, which involved placing non-dispersive infrared multi-gases sensors in a sealed tank, where real commercial batteries with one open end could be settled for operation.
The researchers presented a novel approach for the continuous monitoring of the internal gases of practical commercial LIBs. Various non-dispersive infrared (NDIR) gas sensors were used in a sealed tank to measure the concentrations of CH4, CO2, and C2H4 during battery operation in a typical design.
Based on the characteristics of non-dispersive infrared (NDIR), this technology enabled the rapid operando gas monitoring of real commercial batteries, regardless of whether they were pouch, cylindrical, or prismatic cells. Furthermore, this methodology was used to capture the quantitative consumption of CO2 throughout the discharge process in real batteries, as well as the interaction between CO2 and Li2CO3.
The team developed a model for separating the effects of voltages and temperatures on oxygen development. The role of voltage in CO2 evolution was evaluated. Furthermore, the evolution behaviors of CH4, CO2, and C2H4 were quantified, and the impact of operating temperature variation on the link between CH4 and C2H4 was illustrated. The concentration and rate of CO2 evolution in the electrolyte were also modeled near the electrolyte edge (point V).
Observations
The concentration of CO2 produced closely correlated with both voltage and temperature, but CH4 and C2H4 concentrations were purely reliant on temperature. The concentration of CO2 was larger, and the evolution rate was faster when the temperature was raised to 40°C, and the upper cut-off voltage was raised to 4.5 V. During the second discharge process, C2H4 was consumed, and the concentration dropped below 6500 ppm. The concentration of C2H4 remained stable at 18000 ppm until the solid-electrolyte interphase (SEI) was stable, but CH4 increased to 60400 ppm. When the cut-off voltage was increased to 4.5 V, O2 showed a similar diffusion behavior. Under 4.5 and 4.6 V, the rate of CO2 evolution changed substantially, and the value approached 2 mol m-3 s-1.
More from AZoM: Innovating for the Earth - World Earth Day 2022 on AZoM
At 0.1 s, the rate of O2 creation was around -0.359 mol m-3, whereas the rate of CO2 generation was around -182.713 mol m-3. The CO2 concentration and generation rate peaks occurred at 1.25 and 2.75 seconds, respectively. The existence of Li2CO3 was indicated by a peak near 55.2 eV in the 1s spectra. In C 1s spectra, a prominent peak at about 290 eV was reported as Li2CO3. The peaks between 531.8 and 532.9 eV in the O 1s spectra were assigned to C=O of Li2CO3 and C–O, respectively.
CO2 was linked to O2 creation from a LiNi0.5Mn0.3Co0.2O2 positive electrode as a typical trace gas, which signified stable CO2 release below a threshold voltage of 4.5 V. During the discharge process at different temperatures and cut-off voltages, a higher amount of Li2CO3 was seen on the surface of the graphite-negative electrode.
Conclusions
In conclusion, this study elucidated a unique approach based on the usage of multiple NDIR gas sensors that can be utilized to monitor the internal gas concentration of commercial LIBs in real-time without affecting the battery's performance. The temperature was found to have no effect on O2 diffusion or CO2 concentration. High voltage, on the other hand, accelerated the electrochemical reaction rate at the electrode-electrolyte interface, resulting in a rise in CO2 concentration.
The authors mentioned that such a method might be utilized to see the internal gas evolution in batteries and aid in the understanding of the gas revolution mechanism. They believe that the proposed operando techniques promise to provide a foundation for a better understanding of the interaction of side reactions related to gas evolution between positive and negative electrodes in commercial LIBs.
Disclaimer: The views expressed here are those of the author expressed in their private capacity and do not necessarily represent the views of AZoM.com Limited T/A AZoNetwork the owner and operator of this website. This disclaimer forms part of the Terms and conditions of use of this website.
Source:
Zhang, J., Zhang, X., Bi, S., Rapid operando gas monitor for commercial real-size lithium ion batteries: Gas evolution and relation with electrode materials. Journal of Energy Chemistry (2022). https://www.sciencedirect.com/science/article/abs/pii/S2095495622002005