A paper recently published in the journal Energies reviewed the recent developments in hydrogen storage systems and batteries.
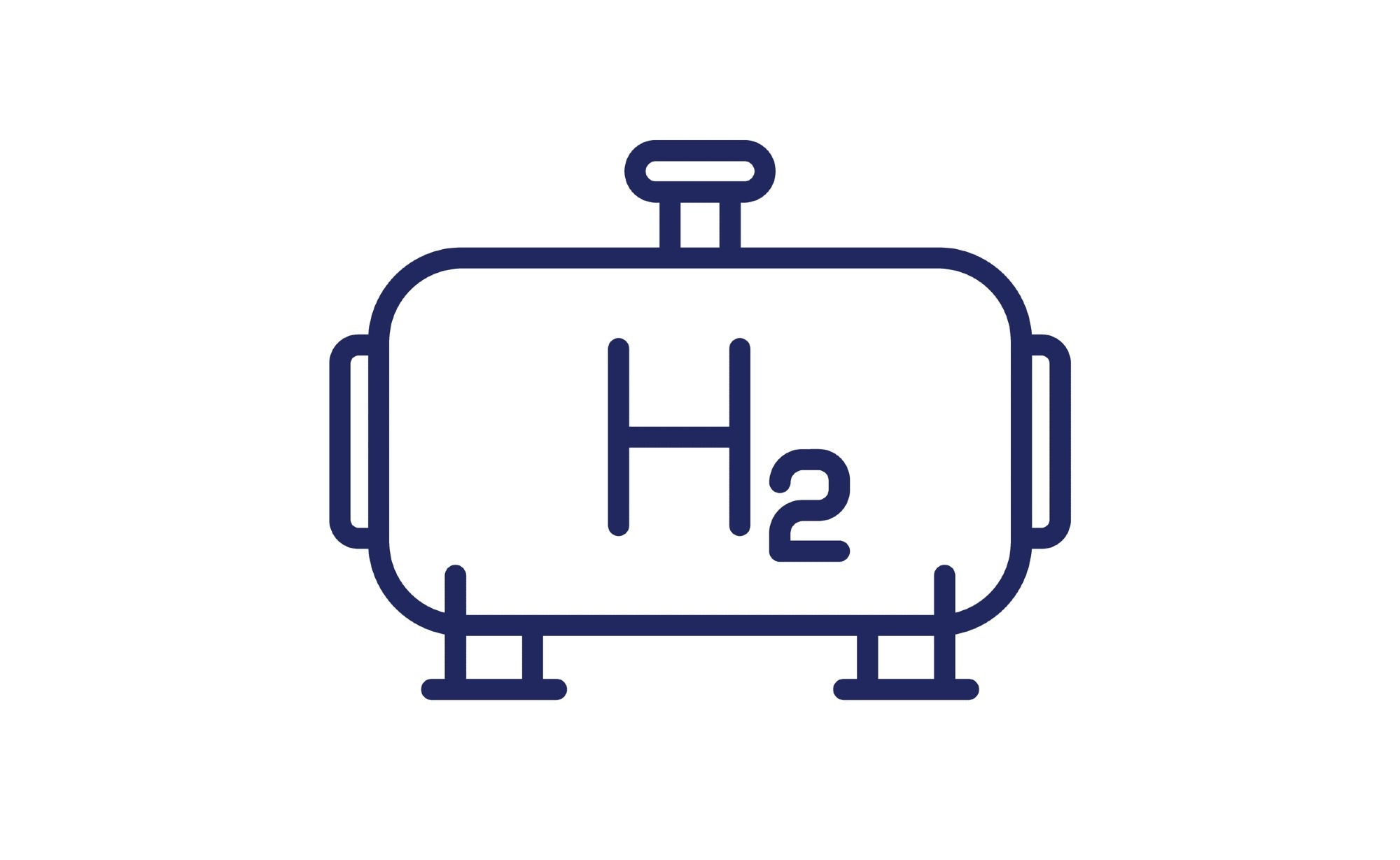
Study: Batteries and Hydrogen Storage: Technical Analysis and Commercial Revision to Select the Best Option. Image Credit: nexusby/Shutterstock.com
Background
Currently, hydrogen storage systems and batteries are the most feasible options for storing energy. These two energy storage methods are either treated as complementary or substitute technologies depending on the energy storage application.
Batteries require lower maintenance, are easy to operate, and possess higher energy capacity, while hydrogen storage systems have better gravimetric and volumetric densities. However, hydrogen storage systems require either low or high temperatures for operation.
In this study, researchers reviewed the current technologies involving hydrogen storage systems and batteries and their commercial applications to understand the evolution of these energy storage technologies thoroughly.
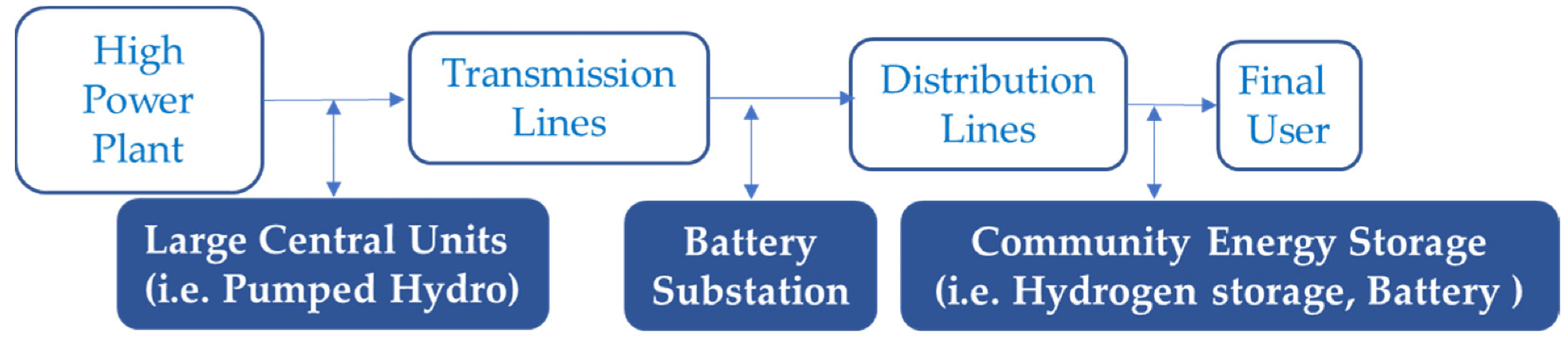
Conventional path of utility energy storage. Image Credit: Andújar, J.M. et al., Energies
Batteries
Conventional batteries, molten salt batteries, redox flow batteries, and the most recent metal-air batteries are the major battery technologies used for energy storage.
Conventional Battery Technology
Conventional batteries, such as Lithium-ion (Li-ion), nickel-cadmium (Ni-Cd), and lead-acid batteries, are used extensively in several commercial applications. In all conventional batteries, a redox reaction occurs in which one of the electrodes releases electrons that are used for supplying load in the external circuit and then carried to the other electrode.
Although lead-acid batteries are used in hybrid electric vehicles (HEVs), submarines, and aircraft, these batteries have several issues with their power performance and specific energy. Ni-Cd batteries require low maintenance compared to lead-acid batteries as they possess a higher corrosion resistance.
These batteries are currently utilized in portable electronics applications. However, the high initial costs and the use of toxic materials to produce Ni-Cd batteries are the major challenges of using this battery technology.
Rechargeable Li-ion batteries are the most efficient conventional battery technology with a relatively long service life. These batteries are commonly used in electronic and medical devices, such as pacemakers. However, Li is an expensive material, and its production is geographically unsustainable, which necessitates the development of suitable and cheaper alternatives such as sodium batteries with similar energy capacity as Li-ion batteries.
The life cycle of Li-ion batteries ranges from 1000-10,000 compared to 500-2500 and 2000-2500 in lead-acid batteries and Ni-Cd batteries, respectively. Moreover, Li-ion batteries also demonstrate a higher round-trip efficiency and have the highest specific energy compared to other conventional batteries.
Molten Salt Batteries
The operation of molten salt batteries, such as sodium-sulfur batteries and ZEBRA batteries, depends on the sodium ion conductivity of more than 0.2 S/cm at 260 °C. Thus, these batteries are typically used in applications where the temperature ranges from 270 °C to 350 °C.
In both ZEBRA and sodium sulfur batteries, a ceramic electrolyte prepared from alumina (β‐Al2O3) transfers the sodium ions between the negative and positive electrodes. Molten salt batteries demonstrate a high efficiency due to no loss of charge and are suitable for application in EVs.
Additionally, they have significantly higher specific energy and lifetime compared to conventional batteries. However, the need for high operating temperatures in molten salt batteries is a major disadvantage as it reduces the battery efficiency and creates self-discharge issues.
The life cycle of sodium sulfur batteries ranges from 2500-4500 compared to 2600-4000 in ZEBRA batteries. Additionally, sodium sulfur batteries demonstrate a higher round-trip efficiency and possess more specific energy compared to ZEBRA batteries.
Redox Flow Batteries
In redox flow batteries, the reactants are dissolved in the electrolyte solution and stored in external tanks. Subsequently, additional balance‐of‐plant devices such as pumps are used to make the stored electrolyte flow. The lifetime of these batteries is not affected by the depth of discharge as conventional rechargeable batteries, which is a major advantage.
Zinc bromide (ZNBR) batteries, vanadium (VRB) batteries, and iron-chromium (ICB) batteries are the major redox-flow batteries currently used for energy storage applications. Among them, the life cycle was highest in VRB, while the round-trip efficiency and specific energy were highest in ICB and ZNBR, respectively.
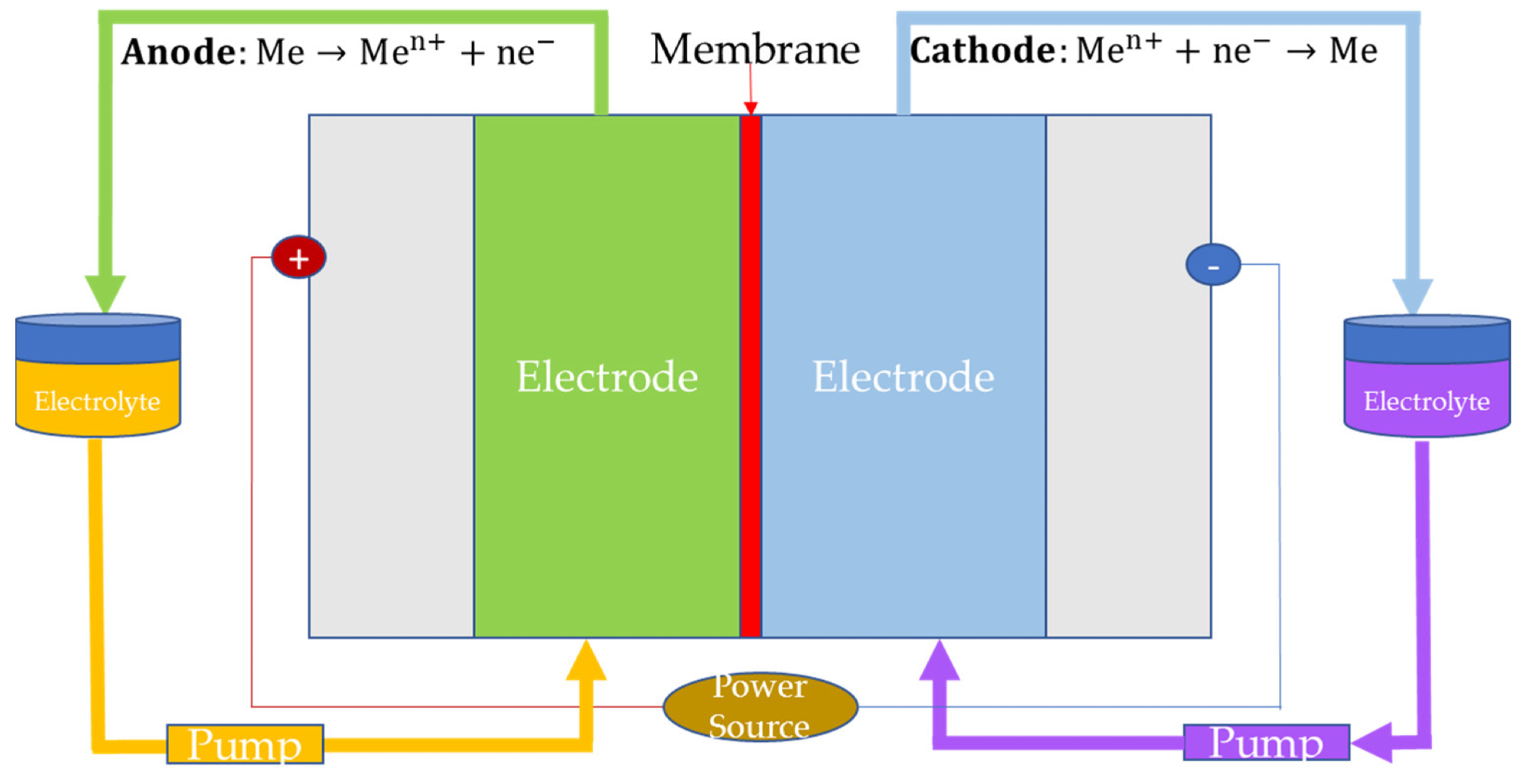
Cell configuration of redox flow batteries (“Me” refers to reactant dissolved in the electrolyte solution). Image Credit: Andújar, J.M. et al., Energies
Metal-Air Batteries
Metal-air batteries were developed to realize batteries with higher specific energy and require lower maintenance. These batteries consist of an open cell structure, with a zinc/Li electrode on one side and an oxygen electrode on the other side.
Zinc-air batteries and Li-air batteries are the prominent secondary metal-air batteries. Although these batteries demonstrated high potential, several issues with the air cathode and metal anode and the short lifetime of the batteries have made them unsuitable for practical applications, such as electrochemical energy storage or EVs.
Among the four battery technologies, conventional batteries demonstrated the maximum round-trip efficiency, while metal-air batteries and redox-flow batteries have the highest specific energy and life cycle, respectively.
Hydrogen Storage Systems
Hydrogen is used as an energy carrier owing to its higher lower heating value (LHV) of 120 MJ/kg compared to other fossil fuels. However, the low volumetric density of 0.0899 g/L of hydrogen at ambient temperature and pressure is a major drawback of using hydrogen storage systems.
The density is substantially lower than the volumetric density targets set by the United States (US) Department of Energy (DoE) and the European Union (EU) for hydrogen storage. Several techniques, such as compressed hydrogen storage, liquid hydrogen storage, and metal hydride storage, were developed to realize the volumetric density required for hydrogen storage.
Compressed Hydrogen Storage
Currently, compressed hydrogen storage is the most commonly used hydrogen storage method. In this method, the hydrogen gas is pressurized at a specific pressure in a metal-composite tank. The pressure can range from 200 bar to 700 bar depending on the use of the hydrogen and the tank.
This method can be used to frequently and repeatedly discharge and charge the hydrogen in the tank for up to 20 years. Higher pressures are typically used to realize volumetric densities that are close to that of liquid hydrogen/ 70.8 gL-1.
Type IV and type III tanks are used extensively to store hydrogen owing to their fatigue resistance, lightweight, and higher nominal working pressure of 350 to 700 bar compared to type II and type I tanks.
Thus, these tanks are often used for industrial purposes or in the automotive sector. Compressed hydrogen storage can be used as small-scale energy storage in buses and cars or as a storage system in microgrids.
Liquid Hydrogen Storage
Although a considerably high volumetric density of 71 g/L can be obtained in the liquid hydrogen storage technique, dealing with the boiloff ratio, which can be 0.1-0.2% of the total stored liquid hydrogen per day, is a significant challenge.
Moreover, the hydrogen critical temperature of 33 K must be attained to obtain liquid hydrogen, which further increases the complexity of the method. Liquid hydrogen storage has already been used in marine applications and aerospace engineering as a temperature sensor.
Metal Hydride Storage
Metal hydrides can be used as hydrogen storage materials in different applications at ambient temperature and pressure. In this method, metal hydrides absorb the solid-state hydrogen molecules to form metallic hydrogen compounds, where the hydrogen is compactly distributed throughout the metal lattice.
The reaction exists as an equilibrium state under a certain temperature and pressure and can be reversed based on requirements. Metal hydride hydrogen storage systems display a maximum round-trip efficiency of 98.94%.
Magnesium, vanadium, iron, chromium, tin, manganese, aluminum, cobalt, and nickel can be combined with hydrogen in this storage method. Metal hydride storage can be used in several mobile and stationary applications, such as railroad, automotive, and heat storage.
Complex Hydrides Storage
Complex hydrides are primarily solid ions consisting of cations covalently bonded to complex anion groups centered on nitrogen, boron, or aluminum and contain hydrogen. Metal hydride decomposition occurs at high temperatures, while the hydriding reaction takes place at high pressures.
The gravimetric and volumetric densities of hydrogen differ in this storage technique based on the material used. For instance, high gravimetric and volumetric densities were obtained when ammonia borane was used to store hydrogen. However, thermal management issues during refueling are the major drawback of this method.
Other Hydrogen Storage Methods
Although the other hydrogen storage methods, such as physisorption and alkalimetal + water have demonstrated considerable potential, these methods are not used extensively in commercial applications.
Among the discussed commercial hydrogen storage technologies, complex hydride storage and compressed hydrogen storage demonstrated the highest volumetric density and round-trip efficiency, respectively. Additionally, both complex hydride storage and liquid hydrogen storage have the highest life cycle.
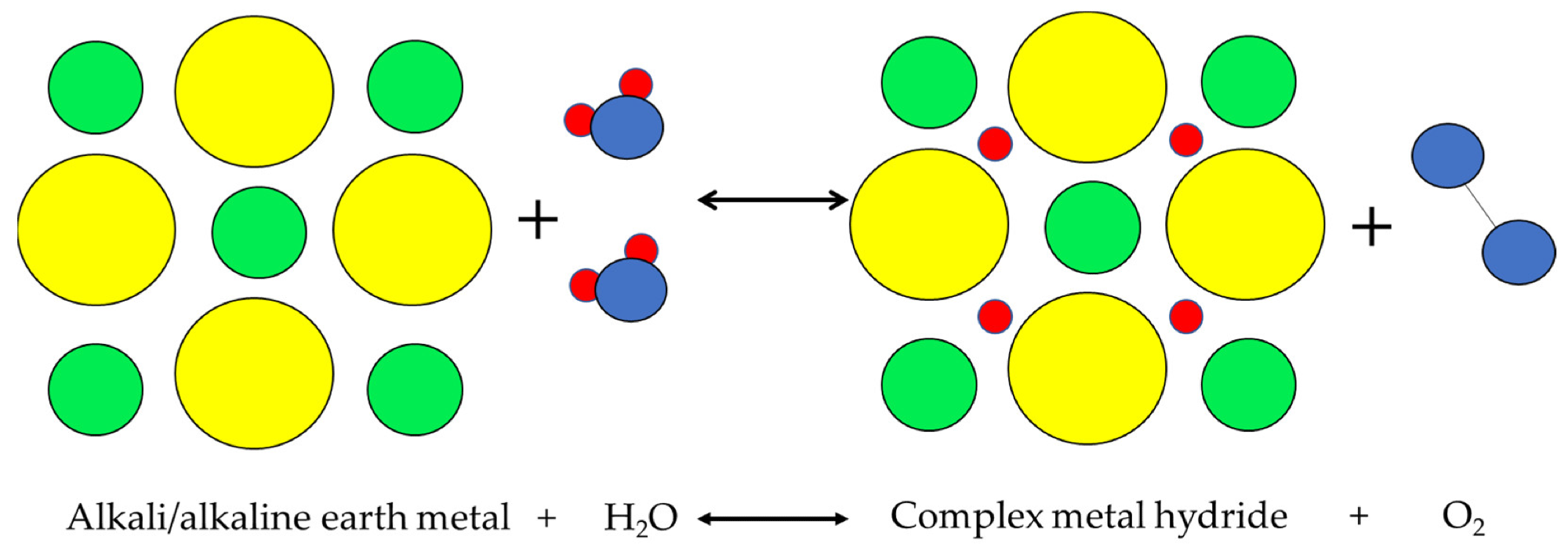
Alkalimetal+H2O physical process. Image Credit: Andújar, J.M. et al., Energies
Conclusion
After analyzing the two major green energy storage systems, researchers concluded that hydrogen storage systems could effectively complement the batteries in different commercial applications in the future. For instance, hydrogen can be used in renewable source-based energy generation plants as long-term storage systems, while batteries can be used as medium- and short-term storage solutions.
Disclaimer: The views expressed here are those of the author expressed in their private capacity and do not necessarily represent the views of AZoM.com Limited T/A AZoNetwork the owner and operator of this website. This disclaimer forms part of the Terms and conditions of use of this website.
Source:
Andújar, J.M., Segura, F., Rey, J. et al. Batteries and Hydrogen Storage: Technical Analysis and Commercial Revision to Select the Best Option. Energies 2022. https://www.mdpi.com/1996-1073/15/17/6196