Scientists from Osaka University and Nara Institute of Science and Technology have reported the development of novel NdNiO3 (NNO) thin films with tunable proton diffusion within their perovskite lattices. Their research has appeared in the journal ACS Applied Electronic Materials.
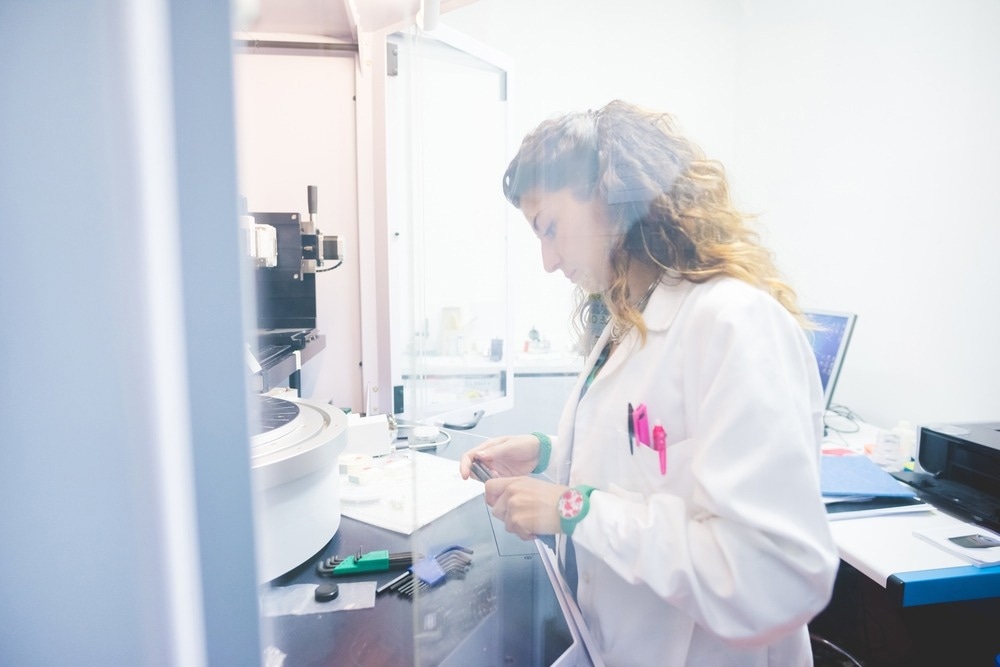
Study: Tunable Proton Diffusion in NdNiO3 Thin Films under Regulated Lattice Strains. Image Credit: Image Source Trading Ltd/Shutterstock.com
Background to the Research
3D-correlated systems possess vast application potential in numerous innovative research fields. These systems display complex electronic phase transitions, which makes them intriguing candidates for technologies such as cognitive computing, photonics, logic-memory systems, and high-performance energy conversion devices.
This property of these systems can be attributed to metal-insulator transition phenomena. Metal-insulator transition is extremely sensitive to carrier/ionic doping and the electrons’ orbital occupancy. Several ionic species can be used to dope these systems, with proton doping being an intriguing approach due to the high ion mobility and small radii of protons.
External electrical fields can be applied to protons to control their diffusion and distribution, and this facile approach can be used to construct a controlled-ionotropic device. Highly tunable and sensitive, these novel devices function based on the principle of proton motion. Proton doping can help overcome the current limitations of semiconductor devices.
In 3D-correlated systems, proton interaction is a particularly complex phenomenon due to the interplay between degrees of freedom, such as the system’s correlated electronic state. Perovskite RNiO3 has garnered widespread interest for correlated ionotronics device applications because of its non-volatile and reversible proton-induced resistivity modulation.
Several studies have investigated this perovskite material for this technological application, with significant research on uncovering the relationship between natural thin-film conditions, lattice strain, and the proton-induced resistivity modulation dynamics.
Bulk NNO has emerged as a perovskite RNiO3 of note in recent studies. This orthorhombic perovskite-structured material possesses some key benefits over previously reported RiNO3 perovskites such as SNO such as a significantly lower thermally driven MIT temperature. Tuning the biaxial strain of films comprised of bulk NNO has potential benefits, such as influencing proton diffusion during catalytic doping.
The Paper
The authors behind the paper in ACS Applied Electronic Materials have produced novel photon diffusion tunable NNO thin films for use in advanced electronic devices. Their approach employs the Pt-catalytic effect to examine hydrogenation-induced metal-insulator transition in these materials.
The Pt-catalytic effect causes hydrogen molecules to dissociate and produce electrons and protons. This effect allowed the team to investigate proton doping behavior upon resistance modulation. Thus, the researchers were able to demonstrate the relationship between tunable proton doping and resistance modulation as a function of the regulated lattice strain in this perovskite system.
Pulsed laser deposition was employed to deposit the NNO thin films, with the deposited films then masked with mesh-like patterns using photolithography. RF sputtering was used to deposit platinum on the thin films, which formed catalyst pads. Dry etching removed the film’s unmasked regions. The remaining resist was removed by submerging samples in acetone.
The resulting NNO thin films were analyzed and categorized using X-ray diffraction, with a physical property measurement system employed to measure the films’ temperature-dependent resistivity. Films were hydrogenated in H2. Proton diffusion was examined using optical microscopy.
Study Findings
The study successfully demonstrated the use of lattice strain regulation to fine-tune proton doping in NNO thin films. The crucial role of strain engineering in enabling proton diffusion doping in RNiO3 perovskites was revealed. The computational analysis supported the author’s innovative experimental approach and confirmed the key role of strain-controlled proton diffusion in determining resistance modulation.
Another key finding in the paper is the enhanced effectiveness of compressive strength for distributing protons in the NNO lattice compared to tensile strain. Lattice strain regulation has the potential to finely tune proton doping behavior and resistance modulation.
Interestingly, after hydrogenation, the film’s lattice constantly changes leading to an unavoidable change in the perovskite’s structure during proton incorporation and transient diffusion, such as elongation. The authors revealed that the substrate-dependent constraint plays a key role in controlling the resulting perovskite structural changes and corresponding properties. Adjusting the substrate conditions can fine-tune the bulk NNO perovskite structure.
In Summary
Overall, the paper has unveiled several key effects of their proton-doping approach on NNO thin film perovskite lattice structure, properties, and characteristics. Strain engineering is a potentially beneficial approach for fine-tuning the proton diffusion in these systems, and approaches such as the modification of substrate conditions can also be advantageous.
The strategy presented in the research provides a potentially powerful route for designing and manufacturing multifunctional controlled ionotronic devices, and the research provides an important contribution to the field. Future research into engineering thin film properties to produce innovative devices with controlled proton motion could provide further improvements.
More from AZoM: How Far Can Semiconductor Manufacturing Be Automated?
Further Reading
Sidik, U et al. (2022) Tunable Proton Diffusion in NdNiO3 Thin Films under Regulated Lattice Strains ACS Applied Energy Materials [online] pubs.acs.org. Available at:
Disclaimer: The views expressed here are those of the author expressed in their private capacity and do not necessarily represent the views of AZoM.com Limited T/A AZoNetwork the owner and operator of this website. This disclaimer forms part of the Terms and conditions of use of this website.