A paper recently published in the journal Small Structures reviewed synthetic strategies and applications of hierarchically porous metal-organic frameworks (HP-MOFs).
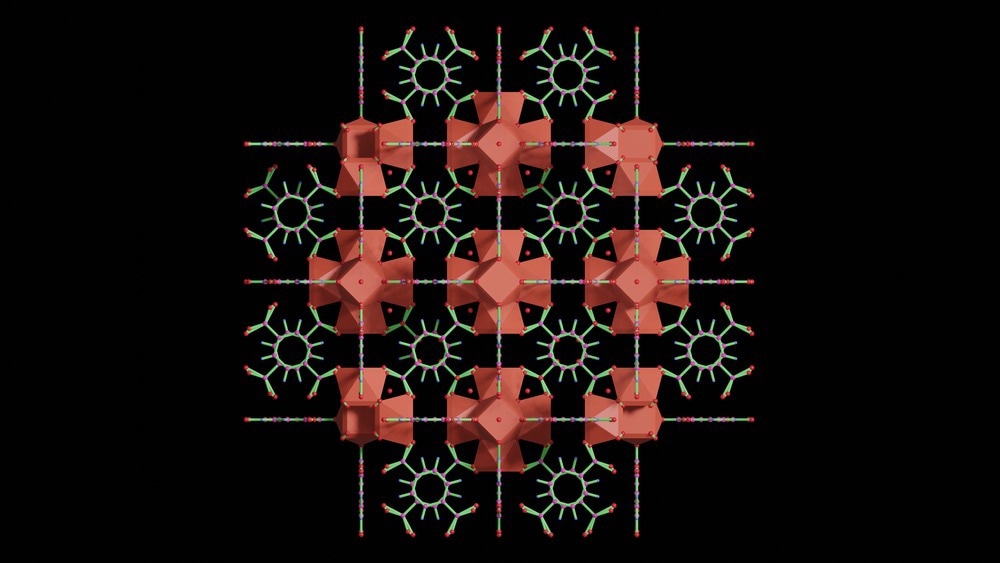
Study: Hierarchically Porous Metal-Organic Frameworks: Synthetic Strategies and Applications. Image Credit: sanjaya viraj bandara/Shutterstock.com
Background
MOFs are primarily assemblies composed of organic ligands and inorganic nodes, such as metal clusters or ions, that have gained considerable attention from the scientific community due to their exceptional morphological features.
MOFs are different compared to traditional porous materials, such as activated carbon, porous silica, and zeolite, as they possess adjustable pore environments, ordered crystalline structures, and large surface areas, which allowed the development of flexible approaches to custom-design MOF structures.
Custom-designed MOFs possess tailored pore sizes and shapes, chemical affinities, and topological structures for specific tasks. However, the narrow pore size of MOFs has limited their use in several applications, specifically those that involve large molecules.
HP-MOFs that combine large microporous material surface areas with the macro-/mesopore spaces allow diffusivity and accessibility by large molecules. HP-MOFs possess several porosity ranges to form hierarchical systems with interconnected pores and desired properties for specific applications.
In this paper, the authors reviewed the synthesis methods of HP-MOFs that contain pores based on interparticle accumulation, defective pores, and intrinsic pores and their applications.
HP-MOF Synthetic Strategies
HP-MOFs Based on Intrinsic Pores
The topological design approach, metal moiety expansion, and ligand extension are the major synthetic strategies used to design HP-MOFs based on intrinsic pores. In the ligand extension strategy, the organic bridging ligand length is extended in intrinsic MOFs to create mesopores. Mesoporous MOFs are also prepared successfully by enlarging the metal node size in MOFs.
Although these methods are used extensively to create HP-MOFs with intrinsic pores, they require a large manpower and a significant amount of material resources. Moreover, these separation and synthesis processes are very complicated in nature. The topological design approach represents an inexpensive and simple strategy to produce HP-MOF structures with intrinsic pores.
For instance, zirconium-based (Zr)-MOFs with intrinsic hierarchical structures, including NU-1000, MOF-545, and porous coordination network (PCN)-222, feature csq topology. Similarly, Materials of Institut Lavoisier (MIL)-100 HP-MOFs feature an mtn topology, while MOF-818 features an spn topology.
HP-MOFs Based on Defective Pores
HP-MOFs with defective pores are produced by incorporating defects in the ordered microporous MOF skeletons while retaining the original MOF single-crystal nature. Intentional defective HP-MOFs and inherent defective HP-MOFs are the major types of HP-MOFs with defective pores.
The hierarchical pore in the inherent defective HP-MOFs is formed due to incomplete metal node coordination. Synthetic strategies that are used to create inherent defective HP-MOFs include metal-ligand-fragment co-assembly functionalization strategy and modulator-induced defect-formation strategy.
Intentional defective HP-MOFs are prepared by introducing defective pores in the microporous MOFs during the post-synthesis modification or the synthetic process. Post-synthetic modification and templating methods are typically used to introduce defective pores in HP-MOFs.
The post-synthetic modification includes a stepwise ligand exchange strategy, etching strategy, encapsulation and post-processing strategy, and linker labilization strategy, while templating methods include cooperative template-directed assembly, self-template strategy soft template strategy, and hard template method.
Hierarchical Pores Based on Interparticle Accumulation
HP-MOFs with well-ordered interparticle mesopores and intraparticle micropores can be created by reducing the MOF particle size to a certain degree. Nucleation-competition strategy, perturbation-assisted nanofusion synthesis, carbon dioxide (CO2)-directed assembly strategy, solvent etching methodology, and MOF nanoparticle gelation are used most extensively to produce HP-MOFs with interparticle accumulation.
Application of HP-MOFs
The meso-/macropores and micropores in HP-MOFs can facilitate large molecule loading, reduce mass transfer barriers, and provide access to active sites. Additionally, the generation of new active sites during defect formation, doping with nanoparticles, and the incorporation of functional groups can make these frameworks suitable for several applications, including heterogeneous catalysis, sensing, energy storage, biotechnology, gas separation and storage, and water remediation.
Cobalt (Co)(II)-NU-1000, molybdenum (Mo)(VI)-NU-1000, and Mo-NU-1200 HP-MOFs with 2.5–3 nm, 3.1 nm, and 2.2 nm pore sizes prepared using the topological design approach were effectively used for oxidative dehydrogenization, cyclohexene epoxidation, and alcohol oxidation, respectively.
MIL-125 titanium (Ti) MOF with 10-30 nm pore sizes was synthesized using the chelating-free, vapor-assisted crystallization method for aromatic sulfur compound oxidation. Selective etching process and etching strategy were used to prepare hollow mesoporous (HM)-MIL-101 with 3.8 nm pore sizes and MIL-101 MOFs for benzyl alcohol oxidation and styrene oxidation, respectively.
Hard template method and encapsulation and etching process were utilized to create highly ordered and oriented macropores within microporous zeolitic imidazolate framework-8 (SOM-ZIF-8) with 190-470 nm pore size and gold (Au)@ZIF-8 with 160 nm pore size for benzaldehyde conversions and aerobic oxidation of alcohols, respectively.
Co-MOF-74 with 100 nm pore size, nickel (Ni)-MOF-74 with 120-140 nm pore size, and hollow Ni (HNi)-MOF-74 with 5-18 nm pore size were synthesized using the self-sacrifice template strategy, acid-induced etching process, and perturbation-assisted nanofusion synthesis for CO2 separation, CO2 adsorption, and vitamin B12 (VB12) encapsulation, respectively.
ZIF-67 with 2.3 nm pore size and phosphotungstic acid (PTA)@HP-Universitetet i Oslo (UiO)-66(HPU3) PTA@HPU-3 with 12.9 nm pore size were prepared using the soft-template strategy and nucleation-competition strategy for oxidation–Knoevenagel condensation and styrene oxidation, respectively.
The modulator-induced strategy was utilized to create UiO-66(Zr)-FA MOF with 1.5-2 nm pore size and HP-PCN-224 iron (Fe) MOF with 2-35 nm pore size for aldol condensation reaction with methyl-isobutyl ketone and sensing, respectively. PCN-222(Fe) MOF with a three nm pore size synthesized using the topological design approach was used for the adsorptive removal of anionic and cationic dyes.
The solvothermal-induced strategy was utilized to create Ni-benzene-1,3,5-tricarboxylate (BTC) MOFs with 3.02 and 3.98 nm pore sizes for energy storage. MOF nanoparticle-based metal-organic aerogels (MOAs) with 9.3–12.9 nm pore sizes were synthesized using the MOF nanoparticle gelation for dye adsorption.
Conclusion
To summarize, significant progress has been made toward the development of new HP-MOF synthetic strategies and the expansion of functional applications of these frameworks. However, more research is required to develop scalable HP-MOF fabrication strategies for commercial applications. Specifically, existing challenges, such as easy agglomeration of metal catalytic sites and difficulties in adjusting the HP-MOF pore sizes based on demand, must be addressed in the near future.
More from AZoM: How are Graphene Batteries Made?
Disclaimer: The views expressed here are those of the author expressed in their private capacity and do not necessarily represent the views of AZoM.com Limited T/A AZoNetwork the owner and operator of this website. This disclaimer forms part of the Terms and conditions of use of this website.
Source:
Chen, B., Chang, G., Yao, Y. et al. Hierarchically Porous Metal-Organic Frameworks: Synthetic Strategies and Applications. Small Structures 2022. https://onlinelibrary.wiley.com/doi/10.1002/sstr.202200187