The 1980s was a boom time for engineering ceramics. Emerging materials such as silicon nitride and SiAlONs were seen as the shape of things to come in several industries, replacing metallic components on a grand scale. For more than a decade, intensive effort went into these new materials. One goal was to produce an all ceramic car engine. However, such an all ceramic future never materialised as reality failed to match up to expectations. Today, ceramics have an important part to play in conjunction with other materials. They can add particular functionality or provide added benefit to a component, eg as hard wearing surfaces, ultra-hard materials in cutting tools, for corrosion resistance or high temperature protection. As ceramics are not being used in isolation, joining is an increasingly vital technology for the integration of the materials.
Joining of Ceramics
Despite its obvious importance, joining is often neglected during the design process. Many engineers incorporate ceramics into a component as though they were high performance metals, giving little thought to service conditions or joining operations. This can lead to two outcomes, either the part fails and its designers conclude that the ceramic was unsuitable and that metals should be used as before, or an expensive redesign may be required if a ceramic must be used.
Joining Considerations
There are many important issues to be considered alongside joining such as materials selection, best practice and joint design, but this article concentrates on ceramic joining technologies and, in particular, some of the novel ways of producing ceramic/ceramic and ceramic/metal bonds.
Ceramic Joining Technologies
The ceramic joining technologies used today (few of which have been developed specifically for this class of materials) range from simple mechanical attachment such as the compression fit used in spark plugs, figure 1a, through to liquid phase processes such as adhesive bonding and brazing. The thermal protection system for the space shuttle uses adhesives, and the ceramic turbocharger rotor uses brazing, figure 1b. There are problems associated with these processes including processing considerations (such as component size and joining atmosphere), time constraints and costs, which is why the new ceramic joining technologies described in this article are being developed.
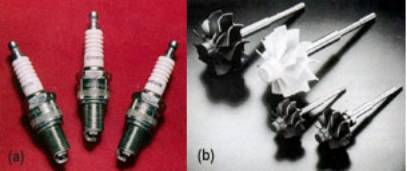
|
Figure 1. (a) Spark plugs manufactured using compression fitting and (b) turbocharger rotor assemblies made from silicon nitride
|
Ultrasonic Joining
Ultrasonic joining, which is used extensively in the plastics industry, has been used for ceramic/metal combinations such as alumina/aluminium, alumina/stainless steel, zirconia/steel and glass ceramic (cordierite-based)/copper. Typical applications include batteries, thread guides, textile cutting equipment and heavy duty electrical fuses. The advantages of the process are the very fast joining times (less than one second), the fact that surface preparation is not critical (contrary to almost every other ceramic joining process) and the lack of melting and intermetallic formation. However, to join hard metals such as steel, soft, deformable interlayers are needed. One limitation of ultrasonic joining of ceramics is that only films or thin sheets of metal can be joined to a ceramic.
The Process
Ultrasonic joining requires a transducer assembly operating at about 20kHz (the source of the ultrasound) coupled to a sonotrode. The sonotrode tip is placed in contact, usually under a clamping load of 1-10Nmm-2, with the workpiece, figure 2. The heat generated is localised at the interface, creating a temperature of up to 600°C when using aluminium interlayers.
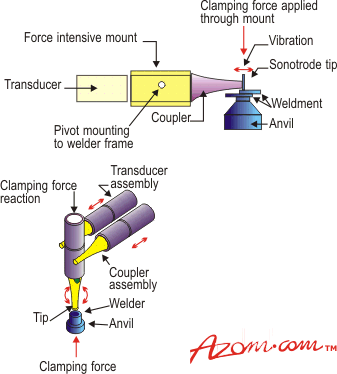
|
Figure 2. An ultrasonic welding set up.
|
The bonding mechanism relies on the vibratory shear stress of the metal exceeding its elastic limit, coupled with the breakdown of surface oxide films exposing atomically clean metal. The clamping force exerts plastic deformation on the metal, which increases the interfacial contact between the metal and ceramic. Mechanical keying then occurs across the interface and the joint is formed, perhaps along with some chemical interactions.
Applications
In future, ultrasonic joining could be applied to gas-tight container seals (lamps), optical components and joining metallic membranes onto ceramic bodies.
Transient Liquid Phase Bonding
Another technique, transient liquid phase bonding (TLPB), has the ability to produce a bond at a lower temperature than that at which it will be ultimately used. The technology is currently being adapted for a number of ceramics using ‘interlayers’ based either on glasses (such as oxynitrides for joining SiAION) or pure metals or alloys (such as Ge and Ge-Si for joining SiC and SiC/SiC composites).
Bonding in the SiAlON system is shown in figure 3. A mixture of silicon nitride, yttria, silica and alumina are applied by spray coating to one surface of the joint. As the samples are heated to 1600°C, a load of 2MNm-2 is applied. Joint formation occurs at this temperature over a period of 10-80 minutes. At about 1400°C, the oxide components react to form a Y-Si-Al-O liquid phase. This leads to densification and sintering. The silicon nitride then dissolves into the liquid, boosting both Si and N contents and altering the composition to Y-Si-Al-O-N. At the same time, (ß-SiAlON grains grow and form an interlocking network across the joint, forming an in-situ reinforcement phase. A secondary process is the diffusion of the adhesive mixture into the surrounding adherent material. Within this diffusion zone the composition and properties of the ceramic gradually change.
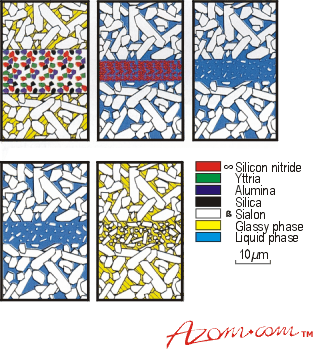
|
Figure 3. The transient liquid phase bonding process.
|
The weakness of the TLPB method is that a favourable reaction between the interlayer and the substrates is required. For silicon nitrides, the glassy interlayer must redistribute itself and penetrate the adjoining microstructure.
Infiltration Processes
High strength, high temperature materials for operation in excess of 1000°C, such as SiC-based composites, are needed for structural applications such as heat exchangers and gas turbine components. This is because traditional stainless steels and superalloys have reached their operational limits. Industry therefore wants to develop a robust joining process suitable for both SiC monolithic and composite materials. Polymers that react with and infiltrate into the bulk material offer a potential solution.
In these so-called infiltration processes, a mixture of polymer precursor (a source of carbon), aluminium, boron and silicon is applied to the joint surfaces (in a tape, paste or slurry) and then heated, generally to 1200°C, in an inert atmosphere and/or air using a propane torch or furnace. The joint forms through pyrolysis of the polymer precursor material, which subsequently reacts with the silicon in the presence of aluminium and boron sintering aids to form in-situ, high density SiC. Strengths range from about 95 MNm-2 for air-joined samples down to 40 MNm-2 for argon atmosphere samples. The fact that the joint can be produced using a simple gas torch could have a major impact on the repair or on-site production of ceramic/ceramic joints. So far, various grades of SiC, SiC/SiC and C/SiC composites have been joined using this technique.
Microwave Joining
Microwaves provide another technique for joining ceramics together. Microwave energy is already being applied to the drying/firing of refractories and whiteware. Now it is being considered as an energy source for joining ceramics such as alumina, zirconia, mullite, silicon nitride and silicon carbide. The direct coupling of the microwave with the ceramic results in volumetric heating, and so there is great potential for heating large sections uniformly. Control of the location of the maximum electric and magnetic fields also enables precise, selective heating.
Conventional diffusion bonding techniques use radiant heating methods and so the time to reach temperature and the time at bonding temperature can be as long as 8 hours. This is particularly the case for materials such as alumina, which are diffusion bonded at temperatures approaching 1600°C. Using a microwave heat source, bonding times can be reduced by an order of magnitude.
Very high purity aluminas are difficult to heat, owing to low inherent dielectric properties making joining difficult. Impure, 85% alumina on the other hand is joined easily. The use of interlayers, including sealing glasses, and alumina gels have both been investigated for producing joints with high purity alumina. Alumina gels offer the advantage that, at the joining temperature, the gel transforms into colloidal α-alumina which subsequently sinters to provide an homogeneous interface. Joints between 85% alumina show bond strengths equivalent to that of the parent material. Joint formation has been studied and a number of possible mechanisms have been identified, depending on the material. For impure aluminas, the glassy grain boundary phase softens and assists in the bonding process, while for zirconia, a solid state process has been identified.
Brazing
Work at TWI is focused mainly on the more traditional methods of joining ceramics, such as brazing, diffusion bonding, glasses and adhesives. However, development programmes are also investigating the modification of braze alloys by the addition of a ceramic reinforcement. This reinforcement provides a joining medium with a coefficient of thermal expansion (CTE) between that of ceramics and metals, and also gives the joint improved strength owing to the introduction of a second phase ie an in situ metal matrix composite. The ability to tailor the CTE of the joining medium is of greater interest. The additional joint strength is a bonus as this raises the possibility of designing the braze to accommodate thermal stresses that would otherwise build up during the joining process.
Sol-Gel
For low temperature applications, novel organic based adhesives reinforced with nano-sized ceramic particles are being fabricated at TWI using sol-gel chemistry, a liquid phase process. This technique allows intimate mixing of the ceramic and organic constituents on a molecular scale, producing materials of high purity and with a high level homogeneity. Sol-gel processing provide a joining medium that is tough yet flexible and can be designed to be hydrophobic and self repairing. Applications for these strong, modified adhesive may be found in the optical, biomedical an defence industries.
Summary
Looking at the field of ceramic joining processes as a whole, techniques have evolved from derivations of metal and plastic joining methods into discrete technologies. However, improvements and modifications to these existing technologies are still required to make them more readily adaptable. In association with this, the selection of the appropriate material and joint design are critical facto when developing joining technologies.
More and more developments in ceramic joining are beginning with a design exercise involving the modelling of new component designs and joint geometries. Modelling is very significant for introducing ceramics into new applications, as it can lessen the number of expensive trial and error failures in material selection, joint design and the selection of the appropriate joining procedure and process cycle.
Modelling
The difficulty faced in the modelling approach is the lack of reproducible material property data for ceramics and joining media such as brazes, solder adhesives etc. If incorrect data is put in, incorrect joint designs will be produced and will give poor support to the decision making process. A great deal of work is therefore concentrating on the development of appropriate data to plug into the models. Until then, the use of modelling for ceramics joint development will continue to be backed up by a series of practical trials.
|