For the spectrochemical study of solid materials with a glow discharge, the Grimm lamp is the geometry of choice. In the late 1960s the initial design was created when Werner Grimm of RSV Präzisionsmessgeräte GmbH in Hanau, Germany developed the lamp.1 This year is the 50th anniversary of this technology’s patent, so it is worthwhile to look at the insight that led to the invention and how it applies today.
Grimm recorded a number of key drawbacks of the spark discharge in his patent filing, and decided to design a source that would not be affected by self-reversal of spectral lines or melt the surface of the sample ending in evaporation and segregation of various components.
Instead, he stated that a number of experiments with hollow cathode glow discharges provided the advantage of “cathodic evaporation” (commonly known as sputtering) in which layers of materials were removed slowly over time.
Yet, it was difficult to switch between samples of interest, and these hollow cathode lamps (HCLs) did not have consistent gas flow through the discharge lamp. HCL emission measurements had minimal adoption barring academic curiosity, in spite of better quantitative results when compared to spark discharges. Grimm’s invention sought to combine the analytical performance of an HCL with the ease-of-use of a spark discharge.
Grimm’s invention placed the tip of the anode in close proximity to the sample cathode without touching it, as illustrated in Figure 1. This meant that the anode was closer than one mean free path from the sample at lower pressure, restricting the chance of an arc being created between the electrodes and allowing the principle drop in potential to happen over an extremely small distance.
.jpg)
Figure 1: Annotated image of Grimm lamp from original patent issued in 1968.1 The anode body comprises most of the discharge cell and a water-cooled cathode plate contacts the sample. A mounting screw holds the sample in place before and after vacuum is initiated. This figure has been slightly modified from the original version.
Grimm would go on to work with glow discharges of various kinds, leading to a number of patents after his initial invention. Which included:
- Use of a Grimm glow discharge for atomic absorption spectrometry
- Application of the Grimm lamp for bulk analysis of wire samples
- Utilization of a glow discharge to polymerize organic compounds
- Analytical optimization for higher sample throughput, more serviceable parts, and greater optical transmission
The final set of studies influenced the commercialization of glow discharges for spectrochemical analysis. Grimm’s suggestions for wire analysis have also been expanded upon and commercially adopted.
Grimm’s company (RSV Präzisionsmessgeräte, GmbH) revealed the first commercially available glow discharge spectrometer in 1979, the Analymat. The resulting product line was purchased by LECO in 1988 and has manufactured glow discharge spectrometers since. The similarities between LECO’s modern Grimm lamp (Figure 2) and the initial invention are remarkable as LECO continues to make use of Grimm’s brilliant insights.
Why the Grimm Lamp Became the Standard Configuration
Glow discharge instruments have continued to be utilized and developed further for spectrochemical analysis of solid materials, as many of the noted drawbacks of the spark discharge carry on today.
One of the primary reasons for the Grimm geometry’s success is the shape of the resulting crater which is made by sputtering material from the sample’s surface and its related characteristics. At best, these craters are flat-bottomed and are a result of the sequential removal of layers of material from the surface.
This crater shape materializes from the very flat electric field with a sharp possible drop over only a few microns between the sample surface (which is the cathode) and an annular anode. Argon ions and atoms are administered toward the surface, which results in even bombardment and sputtering of the sampled area.
The short distance between the anode and the sample cathode is too small for an arc to form and obviates other luminous areas of the discharge besides the analytically useful negative glow.
Step-by-step removal results in the independent examination of virgin material for each period of integration subsequently while sputtering in the same place on the sample for bulk analysis, such as the work carried out by Grimm. These separate integrations are free of metallurgical history from layers nearer to the surface due to the previously sputtered material being removed from the cell. This process also creates data that separates the surface layers from the bulk for characterization that is more accurate.
Glow discharge craters cover less surface area and are shallower than spark spots, which results in less grinding being needed for the preparation of the sample and less reference material consumption. Furthermore, as-cast materials can be examined since graphitic materials are not selectively sublimed in the glow discharge.
.jpg)
Figure 2: Annotated schematic diagram of LECO’s modern glow discharge lamp, with many similarities to the original design by Grimm, including small distances between the end of the anode and the sample cathode, front coupling of the cathode plate to the sample, water cooling in the cathode plate, and the same direction of Ar flow.
Further benefits are apparent in terms of the depth-profiling abilities of these instruments. This potential was shown by a number of researchers, including Boumans2 and Laqua3, within a few years of Grimm’s invention. These primary tests highlighted the significance of knowing the precise power parameters utilized in the discharge and accounting for variations in sputtering rates of materials.
The glow discharge offers several critical advantages for compositional depth profiling when compared to conventional high-vacuum surface analysis techniques, such as:
- More simplicity for non-expert users
- Faster depth profiling analyses
- More sampled area results in greater signal
- Lower vacuum requirements (no turbo-molecular or diffusion pumps needed for the lamp)
- Larger areas that are simultaneously sampled with competitive depth resolution and superior quantitation
How the Grimm Lamp Has Changed
A number of adjustments have been made to adhere to performance and practical sampling goals for users, although the general geometry has remained similar over the last half-century.
One of the main differences between those used in modern instruments and the lamp built by Grimm, are the reamer and facer assembly that cleans the source automatically between analyses.
This feature almost eradicates sample carryover and takes away the requirement to manually clean the electrodes as commonly done with other plasma sources.
The reamer is also employed to keep samples in place, thus removing the need to manually use the mounting screw and bracket shown in Figure 1. An argon blanket has been introduced to allow an argon-rich environment around samples when they are mounted initially, this is to minimize the volume of atmospheric gas that enters the discharge chamber.4
The introduction of radio-frequency power for the analysis of non-conductive samples has been a major advance in glow discharges that has led to some of the most dramatic changes in lamp architecture. Lamps utilized for these samples needed to be adjusted so they have smaller powered areas.
LECO’s RF lamp design most closely mimics the Grimm source, as it minimizes the required capacitive coupling to bias the surface of a nonconductive sample. It includes only a small ring electrode around the O-ring to power samples as shown in Figure 3.5. Other efforts have kept a few elements of the Grimm lamp, like the close placement of an annular anode, but they are different in the way they apply power to the opposite side of the sample.
.jpg)
Figure 3: Photographs of LECO’s DC (left) and RF (right) discharge lamps. The DC lamp still has many similar features of the original Grimm design while the ring electrode on the RF lamp is used to power nonconductive samples for analysis.
Additional changes in lamp design were helpful to users of the instruments. For example, it is vital that users possess more easily replaceable anodes, which wear over time. So the anodes on LECO lamps are unscrewed easily for replacement.
For more robust operation, commercial instruments include replaceable ceramic sleeves to separate the electrodes. Grimm’s original design depended on very close spacing between the outer diameter of the anode tip and the cathode block, with minimal physical insulation.
Some fine adjustments have also been made, anode depths were recorded by Grimm to be optimal at 0.2 mm, but modern instruments are optimized to run with depths nearer to 0.12 mm so arcing is minimized.
Grimm suggested analysis parameters up to 500 mA, 3000 V, and 20 Torr. Today the maximum power parameters are typically around 50 mA and 1200 V with pressures in the range of 5 to 15 Torr.
Modern lamps have been optimized to have a 4 mm diameter area following the introduction of depth-profiling analyses when such a diameter was shown to generate a more optimal flat-bottomed crater (see Figure 4), whereas Grimm’s original lamp had a sampled area with a diameter of 6 mm.
Controlling the Lamp is Critical
Early studies by Boumans2 showed that voltage and current are essential parameters for Boumans’ calculation of sputter rate and quantitation of emission. Over the past thirty years, more attention has been paid to monitoring and control of the discharge parameters in the Grimm lamp. Equation 1 shows the calculation for sputter rate (Q), where CQ is a material-dependent sputtering constant, V0 is a threshold potential for sputtering to occur, and ig and Vg are the discharge current and voltage, respectively.
Q = CQ • ig • (Vg - V0) Equation 1
Without accurate characterization of sputter rate and power, quantitation is impossible. Two samples with the same concentration of a given element will have emission proportional to their sputter rate. When layered materials of disparate composition are analyzed (Figure 4, left), appropriate quantitation can only be obtained with sputter-rate corrected calibration curves.
Such calibrations rely on the maintenance of similar power conditions throughout analysis to sputter as expected, leading to flat-bottomed craters (Figure 4, right). For bulk analyses, consistent power is needed for samples to fit on a calibration curve.
.jpg)
Figure 4: Depth profiling can deliver high precision measurements of both depth and elemental composition (left), but requires fine control of the discharge parameters to obtain flat bottomed craters (right, cross-section imaged by DSX Digital 3D Microscope).
Lamp monitoring and control are more crucial when RF power is utilized because of many sources of power loss between the lamp and power supply. LECO’s glow discharge team has spent a huge amount of effort to characterize these losses and develop mechanisms for calculating them in real time during analyses to measure and stabilize the discharge parameters.6
The method, known as True Plasma Power® is the fundamental step in LECO’s accurate application of RF power, and is what permits accurate characterization of both composition and depth of nonconductive materials. For a few thin layers, understanding of the initial powering parameters can be utilized to correct the data gathered before complete stabilization in accordance with Equation 1.
References and Further Reading
- Grimm, Werner. U.S. Patent No. 3,626,234. 7 Dec. 1971.
- Boumans, P. W. J. M. Anal. Chem.44.7 (1972): 1219-1228.
- Dogan, M., K. Laqua, and H. Massman Spectrochim. Acta B. 26.10 (1971): 631-649.
- Mitchell, Joel C., and Kim A. Marshall. U.S. Patent No. 5,646,726. 8 Jul. 1997.
- Mitchell, Joel C., and Ted J. Casper. U.S. Patent No. 5,408,315. 18 Apr. 1995.
- Marshall, K. A., et al. J. Anal. At. Spectrom. 18.6 (2003): 637-645.
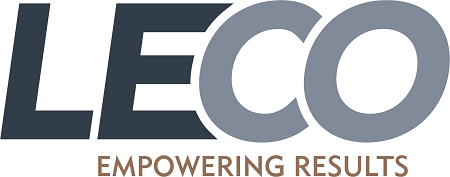
This information has been sourced, reviewed and adapted from materials provided by LECO Corporation.
For more information on this source, please visit LECO Corporation.