With the demand for high-performnce materials increasing, it has become necessary to optimize their structural properties at the nanoscale, underscoring the importance of systematic and routine nanoscale characterization to push forward progress in technology and material science.
The most popular method for such measurements is multimodal scanning transmission electron microscopy or STEM. Conventional STEM and TEM (transmission electron microscopy) techniques include bright field and dark field imaging (or BF/DF STEM) and energy-dispersive spectroscopy (EDS) compositional analysis. These methods are, however, insufficient for full characterization of the underlying structural features that determine the mechanical and/or thermoelectric properties of the studied materials.
Electron diffraction is an efficient technique that can be used to ascertain structural information about sample composition down to the atomic level. Datasets of two-dimensional diffraction patterns (DPs), known as 4D-STEM datasets, can then provide structural information from each individual pixel of the region of interest (ROI).
The development of fast and sensitive pixelated electron diffraction detectors has enabled efficient collection of diffraction patterns in scanning transmission electron microscopes.1 The implemnetation of hybrid pixel technology, resulting in the DECTRIS QUADRO detector, offers high signal-to-noise ratios in 4D-STEM datasets without any risk of detector damage by high currents of the non-diffracted beam.2 At the same time, a large dynamic range (~107) facilitates electron-counting data collection from single electron events to high-intensity diffraction peaks.
The quality of the collected DPs can be significantly improved by using electron beam precession.3 Here, the electron beam is precessed during the collection of a diffraction pattern from each single pixel in the ROI. As the beam is precessed, the signal given by dynamical scattering changes, while the diffraction spots from kinematical scattering do not. Consequently, the signal from dynamical scattering is partially averaged out and the signal-to-noise ratio of the kinematical diffraction spots is increased in the collected DPs.
Beam precession also facilitates excitation of more higher-order diffraction spots, which are then present in the collected DPs. Intensity of all detected diffraction spots is also more homogeneous due to the integration of the signal within the precession angle.
Beam precession can be achieved on conventional TEM/STEM instruments by using the Nanomegas module. However, the technique is constrained by the lack of hardware and software integration in beam precession and direct electron detector readouts, which must be synchronized and aligned independently using different control interfaces.
This article outlines a novel and innovative design of a new analytical STEM microscope, which has been optimized for multimodal, precession-assisted electron diffraction measurements. Here, its capabilities on several selected use cases will be also described.
STEM Microscope with Integrated Beam Precession
The usage and performance of analytical 4D-STEM techniques are usually compromised by the design of conventional TEM/STEM platforms, which were not intrinsically designed for the integration of components needed for fast and high-quality 4D-STEM data acquisition, analysis and processing.1 Such components include a fast and direct electron-diffraction detector, synchronized beam-blanking, electron beam precession, and data processing software for 4D-STEM datasets.
In the new design of TESCAN TENSOR, state-of-the-art components such as a large direct electron detector with hybrid pixel technology and two large EDS detectors have been fully integrated and synchronized with an electrostatic beam blanker and electron beam precession.2 Moreover, a near ultra-high vacuum is provided at the specimen area, resulting in negligible hydrocarbon contamination from the column.
The full integration of all these components facilitates their synchronization and improvement in their performance and overall throughput of STEM, EDS and 4D-STEM measurements. All components can be controlled from a single interface, which provides seamless workflows and a unique user experience.
When the samples are inserted into the microscope and a sample measurement session is started, an overview image of the sample is collected within just a few minutes. After this, users can explore the sample, magnify it and identify the ROI.
After the ROI is selected, the user can choose analytical measurements from a set of predefined workflows. The user needs to set only the key acquisition parameters (such as the beam size, beam current, dwell time and ROI sampling), while the system automatically sets and refines alignments for each measurement. The key microscope alignments, such as focus, astigmatism, speciment height, beam precession and descan pivot points, can be checked and refined manually or by using fully automated functions.
Dedicated hardware have been implemented for beam precession that is used by default at 72,000 Hz. This fast precession rate enables users to run the detector at full speed of 4,500 fps while still providing 16 precession cycles in each frame. Consequently, 4D-STEM datasets can be obtained within minutes and analyzed interactively in the same user interface, instead of an hour or more data collection and later post-processing in external data processing software packages.
The Explore software package also includes processing and evaluation of collected 4D-STEM datasets, which starts already during data acquision. Processed and analyzed results are visualized within minutes, making sample characterization an interactive experience and resulting in enhanced productivity. The Explore approach also makes these advanced electron diffraction techniques accessible for a large range of users without the need of long prior training in using complex high-end TEM/STEM equipment.
For experienced TEM/STEM operators and developers, TENSOR also includes dedicated user interface, ExpertPI, which provides access to all microscope functions and settings at the Python level. These users can customize all measurement conditions and develop their own workflows, including open-source computational packages such as LiberTEM, py4Dstem and HyperSpy.4–6
Deformation Properties of Ni-based Superalloy
Nickel superalloys are advanced engineering materials that can be used in demanding environments such as aerospace and energy generation and are subjected to extreme temperatures, oxidizing conditions, and complex mechanical stress. Understanding these alloys' mechanical properties is crucial for effective and cost-efficient material selection and the optimization of the preparation procedure.
In this example, precession-assisted 4D-STEM automated crystal orientation mapping was employed to analyze crystallographic grain reorientation caused by Vickers indentation-induced plastic deformation at the nanoscale.3 A focused ion beam lamella parallel to the (100) plane was extracted from the plastically deformed region under the indent.
Firstly, a quick summary alignment map acquired from the whole area of the thinned lamella (Figure 1) was used for checking the diffraction pattern quality, refining the diffraction template processing parameters, and for localization of the ROI. The selected ROI (3x3 µm2) with a large orientation gradient under the indentation point was then analyzed at higher magnification using 6.5-nm pixel size.
The high-resolution orientation map (Figure 1) revealed that the areas of heavy slip-plane density seen in BF/DF STEM images correlated with the areas with a large orientation change (the sub-region shown in yellow).
![Overview of a prepared FIB lamella of a Ni-based superalloy extracted from bellow of an indent, shown in the bright field STEM image (top left). A quick orientation map of the entire lamella (bottom left) was acquired to check and optimize diffraction data acquisition settings and to identify the region of interest. A detailed orientation map of the region of interest (right) exhibits significant reorientation from the original [001] orientation (red) to the new [103] orientation (yellow) under the indent due to strong plastic deformation](https://www.azom.com/images/Article_Images/ImageForArticle_23693_17176663903038815.png)
Figure 1. Overview of a prepared FIB lamella of a Ni-based superalloy extracted from bellow of an indent, shown in the bright field STEM image (top left). A quick orientation map of the entire lamella (bottom left) was acquired to check and optimize diffraction data acquisition settings and to identify the region of interest. A detailed orientation map of the region of interest (right) exhibits significant reorientation from the original [001] orientation (red) to the new [103] orientation (yellow) under the indent due to strong plastic deformation. Image Credit: TESCAN Group
Structural Characterization of Battery Electrodes
There is much interest in understanding how microstructural engineering improves the cycling behavior of electrodes and the extent to which experimental conditions can be tuned to produce specific morphologies in materials developed for the next generation of batteries.
In the case of electrodes based on LiTi2(PO4)3, researchers have demonstrated that particles with spindle-like morphology, synthesized by solvothermal reactions, are molded from small sub-particles and influence enhanced battery cycling performance compared to sol-gel synthesized material.7
Previous microstructural evaluation of such particles has shown the presence of two minor secondary phases: TiO2 nanoparticles and a LiTiOPO4 phase. The majority of the TiO2 nanoparticles form a three-dimensional network; however, whether or not these networks exhibit crystallographic ordering is uncertain.8
In this example, precession-assisted 4D-STEM was used to map different phases and reveal individual grains at the nanoscale, based on their crystal lattice orientation, which the conventional techniques, such as high-resolution imaging, TEM-selected area diffraction, and EDX mapping analysis, have not been able to reliably get answer to.
A FIB lamella was prepared from synthesized particles and the 4D-STEM dataset for phase and orientation evaluation was acquired. The kinematic diffraction templates for orientation mapping were generated for the known structures of the TiO2 and LiTi2(PO4)3 phases.
The combination of both phase and orientation maps (Figure 2) revealed that the TiO2 particles were located at the LiTi2(PO4)3 sub-particle boundaries. The LiTiOPO4 phase was amorphous and only found in two small discrete regions as a minority phase.
Understanding the grain boundary behavior and properties will be of significant interest for future research, as the internal sub-particle boundaries can enhance lithium ionic conductivity, improving battery performance.
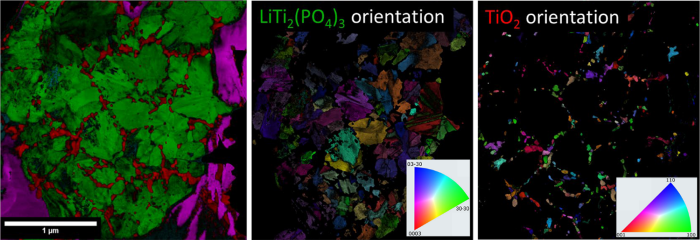
Figure 2. Precession-assisted 4D-STEM phase map (left) of the LiTi2(PO4)3 (green) and TiO2 (red) phases with their respective orientation maps and legends (middle and right). The minority phase of LiTiOPO4 is shown in cyan in the phase map (left). Image Credit: TESCAN Group
Conclusions
The new design of an analytical STEM microscope, TESCAN TENSOR, with fully integrated precession-assisted diffraction measurements has facilitated rapid and intuitive sample evaluation using seamless 4D-STEM workflows.
New understanding of Ni-based superalloys' deformation behaviors and the distribution of titanium and lithium phases in spindle-like battery anode nanoparticles were attained by utilizing the precession-assisted 4D-STEM phase and orientaiton techniques that now become accessible to a broad range of scientists by using TENSOR. This level of sample characterization is not obtainable using standard techniques, such as selected aperture electron diffraction, EDX compositional evaluation, and high-resolution imaging.
Acknowledgments
Produced from materials originally authored by Daniel Němeček from Tescan Group. DN and Tescan are grateful for collaboration on these 4D-STEM application examples with the group of Prof. Rafal Dunin-Borkowski and Dr. Penghan Lu from the Ernst Ruska Center for Microscopy and Spectroscopy with Electrons, FZ Julich, Germany.
References
- Ophus, C. (2019) Microsc. Microanal., 25(3), pp.563–582.
- Stroppa, D.G., et al. (2023) Microscopy Today, 31(2), pp.10–14.
- Vincent, R. and Midgley, P. (1994) Ultramicroscopy, 53, pp.271–282.
- Clausen, A., et al. (2020) J. Open Source Software, 5(50), pp.1–4.
- de la Pena, F., et al. (2017) Microsc. Microanal., 23(S1), pp.214-215.
- Savitzky, B.H., et al. (2021) Microsc. Microanal., 27(4), pp.712–743.
- Yu, S. et al. (2016) Chem. Electro. Chem., 3(7), pp.1157–1169.
- Zhang, Q. et al. (2021) RSC Advances, 11(55), pp.34605–34612.
- Rauch, E.F. et al, (2008) Microsc. Microanal., 22(6): S5–8
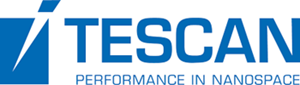
This information has been sourced, reviewed and adapted from materials provided by TESCAN Group.
For more information on this source, please visit TESCAN Group.