This article is based on the webinar delivered by Dr. Craig Wall of Montana Instruments and Dr. Benjamin Pingault of the Laboratory for Nanoscale Optics at Harvard University.
In this article, we’ll look at cutting-edge research into the development of solid-state single-photon emitters (SPEs) for on-chip photonics applications in quantum information science, including the characteristics of silicon and tin vacancy centers in diamond, the challenges of coupling quantum emitters with defect engineering and strain tuning, and spin properties and coherence times for group IV color centers. We will also take a deeper look at the technology that makes this research possible, including a cryogenic, high NA, and low vibration experimental setup.
Single-Photon Emitters are Becoming a Reality
Since the early 2000s, there has been a great deal of interest in the development of non-classical light sources: sources that produce streams of identical photons with controllable quantum correlations. A central building block of these devices is a single-photon emitter (SPE).1 Although the idea of a single quantum of light – a photon – was proposed by Max Planck in 1900, it would be decades until single-photons would be produced and studied.2,3
We can define an ideal single-photon emitter (SPE) as a device that emits indistinguishable photons exactly one at a time into a given spatio-temporal mode. Although a true on-demand ideal photon source has yet to be realized, researchers are successfully using a variety of approaches to make progress towards the ideal SPE that can be an integral part of an on-chip quantum device.4
- Atomic transitions: These sources use trapped ions or cold atoms and are well-controlled. These are typically complex setups, requiring extreme vacuum environments where loading atoms into the trap can be problematic.
- Spontaneous parametric down-conversion (SPDC): These approaches convert a high-energy photon into a pair of identical lower-energy photons.
- Solid-state single-photon emitters, such as point-defects in solids (also known as color centers). These devices will be the main focus of this article.
Color Centers as Solid-State Single-Photon Emitters
A color center is essentially a fluorescent point defect in a solid. Such a defect behaves like a single trapped atom in a solid-state matrix and is stable at room temperature (provided the ground and excited states are far away from that of the host crystal lattice). Nitrogen-vacancy centers in diamond and silicon-vacancy centers have been the most widely studied since both can operate as SPEs at room temperature. However, using a cryogenic environment to lower the temperature to below 5 K greatly improves the zero-phonon line emission and coherence times.
Despite some remaining challenges, color centers have already found various applications, ranging from magnetometry to bio-labeling, thermometry, electrometry, and quantum information processing.
The Potential of Silicon-Vacancy Centers as Quantum Information Carriers
Silicon-vacancy centers (SiV-) stand out from other color centers being targeted for quantum information science applications due to the quality of their optical properties. First, 70-80% of emission is zero-phonon line emission, which is required to use photons as quantum information carriers in practical devices.5
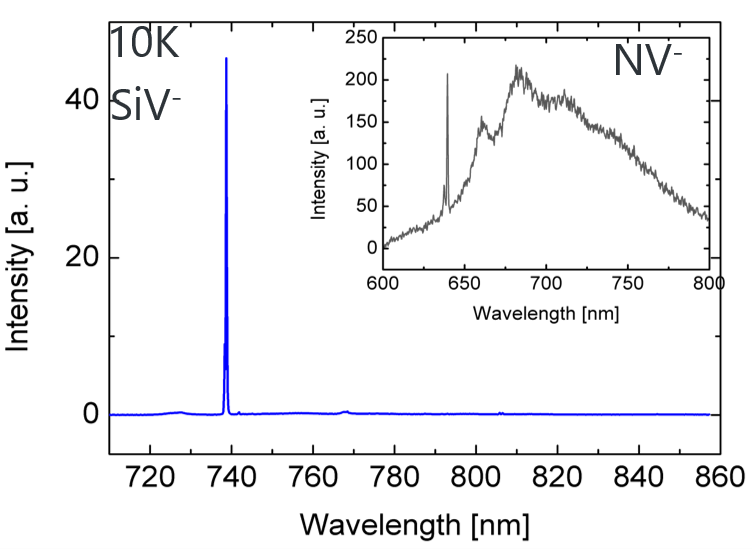
Figure 1. The emission spectrum of the silicon-vacancy center, showing the single-phonon line at 737 nm. Image Credit: Dr. Benjamin Pingault, Laboratory for Nanoscale Optics, Harvard University
Second, SiV- centers are good sources of indistinguishable photons, which is important to interface several SiV‑ centers with each other using photonic means such as waveguides and beam-splitters. 6
Third, SiV- centers are stable in nanodiamond-structures (or at least, more stable than NV centers in similar small-scale structures, for example). Nanodiamonds as small as 1.6 nm can host stable SiV- centers, making them attractive candidates for nanoscale applications .7,8
Currently, researchers are focusing on integrating SiV- centers into a quantum network as optically interfaceable qubits.9,10 So far, researchers have succeeded in coherent control of the spin using only optical coupling or using a combination of microwaves and visible light.11,12 One of the remaining challenges is that the spin-coherence time at 4 K is limited to around 100 ns by phonon-mediated excitations between the two orbital branches of the ground state (Figure 2). Quantum information processing applications often require much longer coherence times.
Increasing Coherence Times of Si-V- Centers Without Lowering Temperature
Potential ways to increase the coherence time include further decreasing the operating temperature (which would require expensive and complex dilution refrigeration setups), altering the phonon density of states at 50 GHz, or splitting the ground state’s orbital branches.13
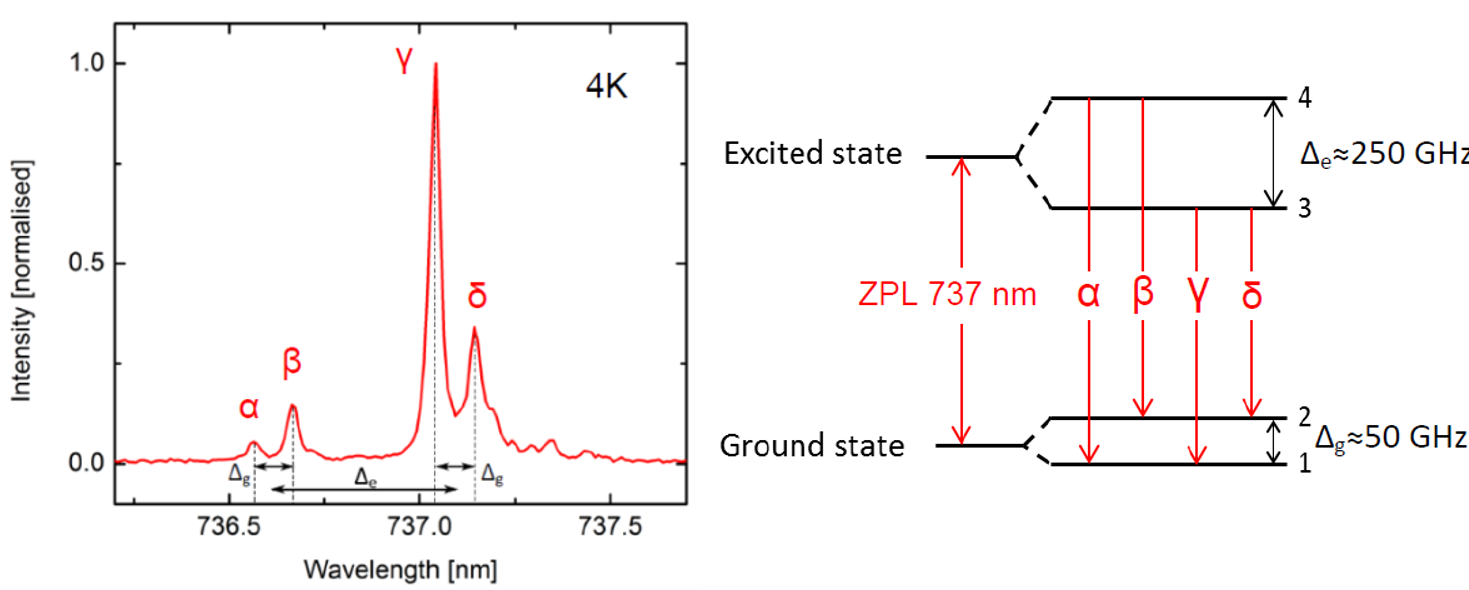
Figure 2. Normalized emission spectrum and state transitions of SiV ‑. Image Credit: https://www.nature.com/articles/ncomms4328
Dr. Pingault and his collaborators at Harvard University split the ground-state orbital branches using tunable cantilevers etched into a bulk diamond with implanted Si and metal electrodes on the top and the bottom. Applying a voltage to the electrodes causes the cantilever to bend, thus reliably applying a tunable strain to a silicon-vacancy.
The application of strain to the vacancy causes the spins to split apart from each other, which corresponds to the splitting of energy levels. In this way, the team increased the ground-state splitting in SiV- from 50 GHz to 470 GHz.14
Using strain-controlled ground-state splitting produced spin coherence times of 250 ± 20 ns and a spin decay time of 2.5 μs at 4 K, comparable to the spin coherence time of 300 ns at 100 mK achieved using dilution refrigeration.13, 14
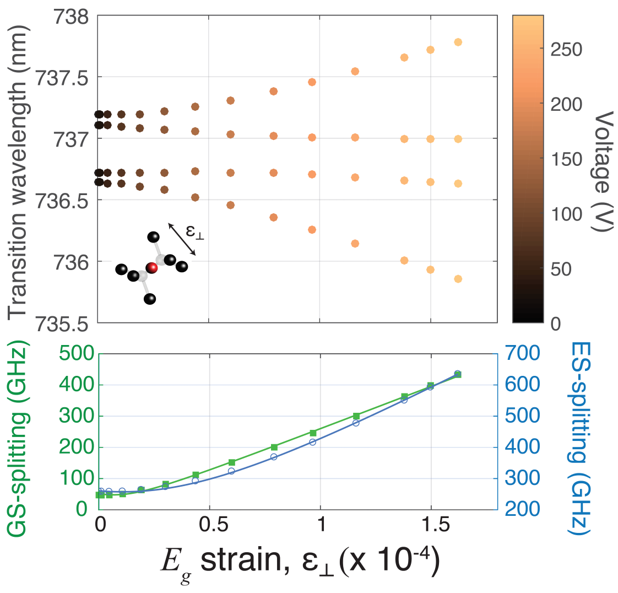
Figure 3. Strain-induced ground-state splitting of SiV -. Image Credit: https://www.nature.com/articles/s41467-018-04340-3
Increasing Coherence Times Using Tin-Vacancy Centers
Ongoing research efforts by Dr. Pingault and his group to achieve increased spin coherence time in color centers indicate that the tin-vacancy center (SnV), with a ground-state splitting of 850 GHz (as opposed to SiV- at 50 GHz), is more amenable to the creation of long spin coherence times. A spin decay time of 11 ms at 3.25 K has been measured, which is one to two orders of magnitude higher than for SiV- or GeV centers in similar conditions.15,16
Practicalities of Characterizing and Tuning Color Center Quantum Emitters
Integrated single-photon emitter experiments like those performed by Dr. Pingault and his team require stringent experimental conditions that are repeatable over many experimental cycles and can be maintained over the course of long-duration experiments (sometimes a month or more at a time). The researchers require a setup with:
- Low temperatures (< 4 K) and high-temperature stability. The low temperatures ensure minimal thermal noise and a higher probability of ZPL emission. An automated setup that can be temperature controlled remotely can greatly improve throughput and feedback on experimental data collection. High-temperature stability keeps the SPE in focus by reducing the amount of drift from thermal expansion/contraction.
- Mechanical stability to minimize vibrations that would decrease color center coherence times, reduce the optical collection efficiency from a high NA objective, or excite unwanted mechanical modes in the integrated device.
- Precise optical fiber positioning. A stable optical fiber is required to maximize the excitation and collection of photons from an integrated SPE device. The fiber often must be positioned with better than 200 nm accuracy and the position should remain stable throughout the experiment.
- High numerical aperture objective to maximize the collection of photons and provide diffraction-limited imaging of the single color centers. Collection efficiency is important for improving the probability of observing the quantum device operation and thus reducing the amount of time required to perform an experiment.
- Microwave electrical contacts can be used to power a strip line used to split energy levels, and a gas tube feedthrough can be used to tune device properties or to tune the resonance of a cavity.
To best realize these experimental requirements, the Montana Instruments Cryostation with a high NA in-vacuum objective lens was used to perform the experiments. The Cryostation is a high-performance optical cryostat engineered to meet the exacting measurement conditions required for quantum materials and quantum information science research. Precise temperature control, low thermal fluctuations, and nanometer level vibrations provide a stable optical and electrical measurement environment maximized for high collection efficiency. Fast cooldowns, automated operation modes over a wide temperature range (3.2 K – 350 K), and remote control provide an ideal tool for color center and SPE research.
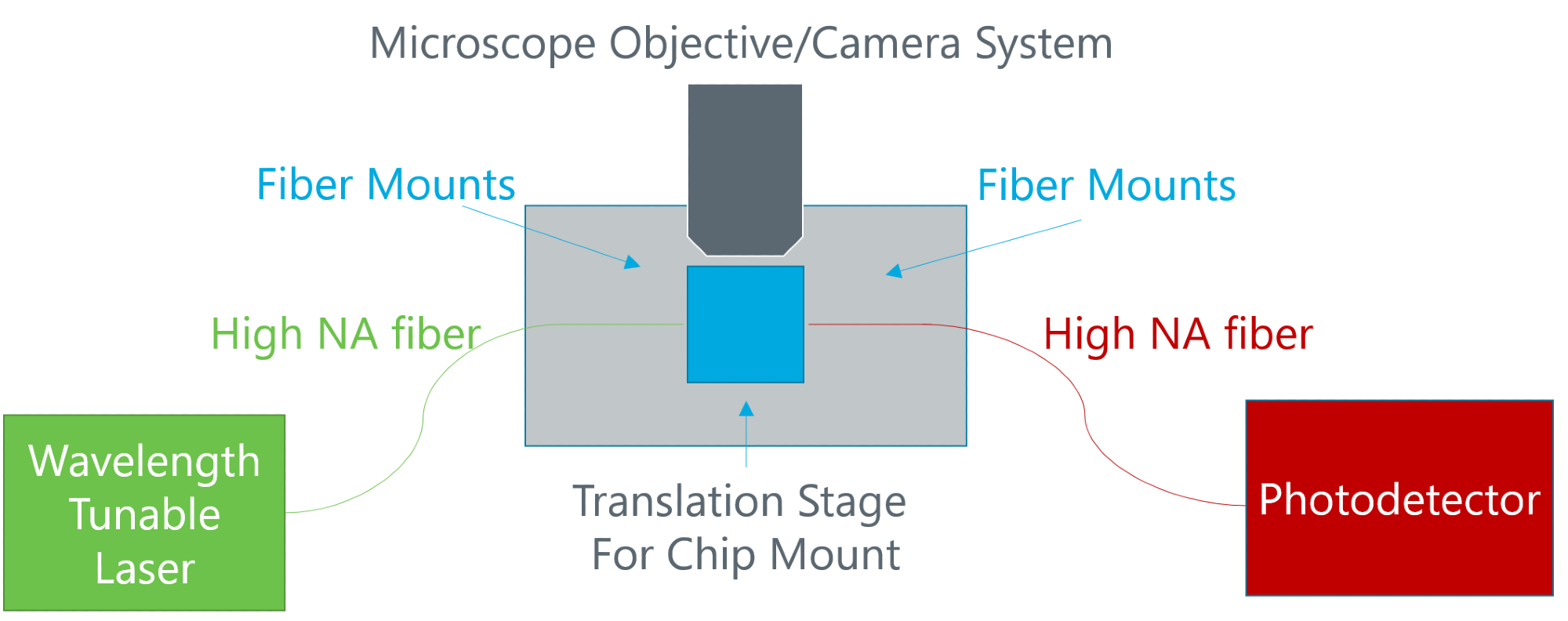
Figure 4. Schematic diagram of experimental set up for characterizing and tuning color center quantum emitters. Image Credit: Montana Instruments
Conclusion
Group IV color centers such as SiV- and SnV centers make promising spin qubits. Their optical properties and large strain susceptibility allow single-photon interfacing of quantum network nodes which is a major step towards practical on-chip photonic integration.
In the full webinar, Dr. Pingault reviews details of the experiments above, demonstrating tunable SPE devices that can be coupled for quantum information science applications. Dr. Wall then discusses the considerations for setting up a cryogenic platform with suitably low vibrations and high numerical aperture that can be used to characterize and tune color centers. To view more detailed results, figures, and example experimental setups, download the full slide deck below. Or, view the full recording of the live webinar here.
Download the Slide Deck here!
References and Further Reading
- Aharonovich, I., Englund, D. & Toth, M. Solid-state single-photon emitters. Nature Photonics 10, 631–641 (2016).
- Planck, M. Über eine Verbesserung der Wienschen Spektralgleichung. in Von Kirchhoff bis Planck: Theorie der Wärmestrahlung in historisch-kritischer Darstellung (ed. Schöpf, H.-G.) 175–178 (Vieweg+Teubner Verlag, 1978). doi:10.1007/978-3-663-13885-3_15.
- Clauser, J. F. EXPERIMENTAL DISTINCTION BETWEEN THE QUANTUM AND CLASSICAL FIELD THEORETIC PREDICTIONS FOR THE PHOTOELECTRIC EFFECT. (1973).
- Meyer-Scott, E., Silberhorn, C. & Migdall, A. Single-photon sources: Approaching the ideal through multiplexing. Review of Scientific Instruments 91, 041101 (2020).
- Neu, E. et al. Single photon emission from silicon-vacancy colour centres in chemical vapour deposition nano-diamonds on iridium. New J. Phys. 13, 025012 (2011).
- Sipahigil, A. et al. Indistinguishable Photons from Separated Silicon-Vacancy Centers in Diamond. Phys. Rev. Lett. 113, 113602 (2014).
- Sipahigil, A. et al. An integrated diamond nanophotonics platform for quantum-optical networks. Science 354, 847–850 (2016).
- Evans, R. E. et al. Photon-mediated interactions between quantum emitters in a diamond nanocavity. Science 362, 662–665 (2018).
- Pingault, B. et al. All-Optical Formation of Coherent Dark States of Silicon-Vacancy Spins in Diamond. Phys. Rev. Lett. 113, 263601 (2014).
- Rogers, L. J. et al. All-Optical Initialization, Readout, and Coherent Preparation of Single Silicon-Vacancy Spins in Diamond. Phys. Rev. Lett. 113, 263602 (2014).
- Pingault, B. et al. Coherent control of the silicon-vacancy spin in diamond. Nature Communications 8, 15579 (2017).
- Becker, J. N. et al. All-Optical Control of the Silicon-Vacancy Spin in Diamond at Millikelvin Temperatures. Phys. Rev. Lett. 120, 053603 (2018).
- Sukachev, D. D. et al. Silicon-Vacancy Spin Qubit in Diamond: A Quantum Memory Exceeding 10 ms with Single-Shot State Readout. Phys. Rev. Lett. 119, 223602 (2017).
- Sohn, Y.-I. et al. Controlling the coherence of a diamond spin qubit through its strain environment. Nature Communications 9, 2012 (2018).
- Iwasaki, T. et al. Tin-Vacancy Quantum Emitters in Diamond. Phys. Rev. Lett. 119, 253601 (2017).
- Single-Photon-Emitting Optical Centers in Diamond Fabricated upon Sn Implantation | ACS Photonics. https://pubs.acs.org/doi/abs/10.1021/acsphotonics.7b00904.
- Cryo-Optic | Montana Instruments. https://www.montanainstruments.com/Products/Cryo-Optic/.
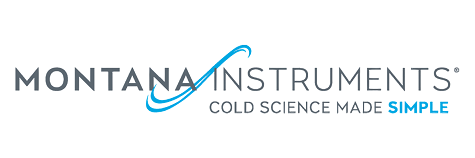
This information has been sourced, reviewed and adapted from materials provided by Montana Instruments.
For more information on this source, please visit Montana Instruments.