Wet-chemistry techniques have been used to create a variety of metal and semiconductor nanocrystals, including Au and Ag nanocrystals with fascinating plasmonic characteristics in the visible-to-near-infrared ranges.
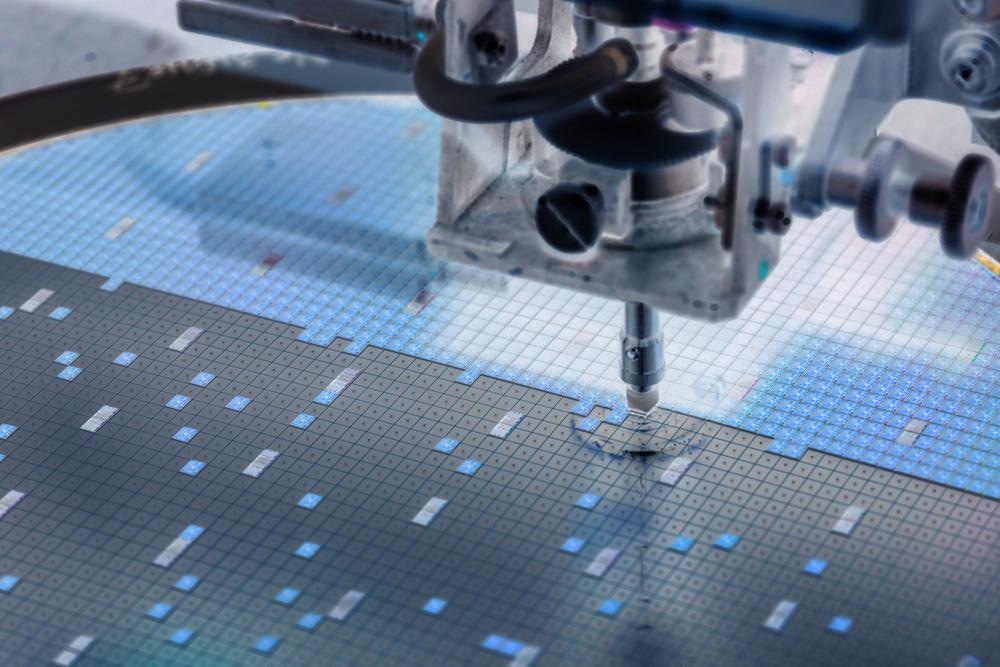
Image Credit: Macro photo/Shutterstock.com
The latest study published in ACS Omega focuses on WO3-Au hybrids and their efficient properties.
Properties of WO3-Au Metal/Semiconductor Hybrid
A simple hydrothermal approach was used to create a WO3-Au metal/semiconductor hybrid, which consisted of a basic framework of WO3 nano brick strengthened by the presence of Au nanoparticle additives. The WO3-Au hybrids offer superior light absorption, robust plasmonic interaction, superior performance SERS, and catalytic properties.
Furthermore, during visible light irradiation, the WO3-Au hybrids display remarkable photocatalytic properties toward RhB breakdown. The robust plasmonic resonance and effective conduction mechanism between Au and WO3 nano bricks are credited with the increased photocatalytic performance. This study would prove fruitful especially for the electronics sector and all other structural industries utilizing semiconductor metal hybrids.
Industrial Utilization
Such metal-semiconductor hybrids have found their applications in many industries from highly technological macroscale industrial applications to nanoscale technological applications. The major ones include Plasmonic applications. These include Plasmonic catalytic reactions, photogeneration of pollutants, photocatalytic Hydrogen synthesis, and photocatalytic CO2 process.
The optical applications are also a major one including Plasmon-Enhanced Photoluminescence and Surface-Enhanced Raman applications. Solar cell applications and biotechnological applications also readily utilize this advantageous substance for their applications.
Limitations
Although many advantages are known, several limitations also act as a hurdle in its rapid commercialization and vast applications. Due to the Lar Mer growth process of high-temperature organic phase synthesis, the metal or semiconductor portion could not be managed in greater dimensions, such as tens to hundreds of nanometers.
Another major problem is the size of the two components; as it is mainly still confined to the 10 nm range, the junction between metal and semiconductor is still not sufficiently managed to be clean enough.
Materials and Methods
The materials used in this experiment included ninety-nine point five percent of Sodium tungstate (99.5%), ninety-nine point seven percent of L-ascorbic acid, hydrochloric acid (30%), and chloroauric acid (99.9%).
The first step involved the synthesis of the semiconductor hybrid. A simple hydrothermal process was used to create the WO3 nano-bricks. A reactor was filled with 20 mL of sodium tungstate (0.01 M) and 6 mL of chloroauric acid. The mixed solution was placed on a hot plate and heated to 180 °C for 24 hours. To eliminate contaminants, the finished products were centrifuged at 5000 rpm for 5 minutes.
For SERS measurements, RhB molecules were placed as substrates on WO3 and WO3-Au hybrids. The substrates were made by combining 1 mL of RhB at various concentrations with 0.5 mL of WO3 and WO3-Au (0.5 mg mL1). The solution was mixed and left in the dark for 2 hours.
The photocatalytic capabilities of WO3 and WO3-Au hybrids were evaluated using RhB photodegradation under the illumination of a 300 W xenon lamp with an ultraviolet exclusion filter.
The TEM pictures were captured using a JEOL 2100 at 200 kV. HRTEM and EDS were carried out using a JEOL 2010 FET microscope with a 200 kV accelerating voltage.
Experimental Findings
The experimental results confirmed that the WO3 had a flat surface and a consistent brick shape. Growing Au nanoparticles on WO3 nano-bricks resulted in Au-decorated WO3 nano-bricks, with chloroauric acid and ascorbic acid serving as the antecedent and reductant, respectively.
The research showed that the Au nanoparticles have a flowerlike shape with an average size of 34 nm. With a lattice spacing of 0.38 nm, the WO3 nano-brick exhibited continuous and orderly lattice fringes. The WO3 pattern corresponded nicely to the triclinic-phase WO3. The topology of the WO3-Au semiconductor/metal hybrids was modified by varying the quantity of chloroauric acid used during the production procedure.

Image Credit: Mike_shots/Shutterstock.com
The bending vibration mode of O-W-O was deemed responsible for the conspicuous peaks at 275 and 328 cm-1. The peaks at 712 and 810 cm-1 are caused by WO's stretching vibration mode. The surface oxygen vacancies on WO3 can offer additional active sites for the adsorption of RhB.
The Raman amplitude of the WO3-Au hybrids at 1647 cm1 was 1.98 and 5.86 times that of Au nanoparticles and WO3, correspondingly. The Raman intensity of WO3- Au (2.1 wt percent) at 1647 cm1 was 10.4 and 3.51 times that of pure WO3 and Au nanoparticles, respectively. The minimum measurable limit is 10-12 M, whereas the highest EF (at 1647 cm-1) is determined to be 5.3*108.
Additional Au nanoparticles can be added to increase the number of interlayer hotspots. The more of the Au nanoparticles that are placed on WO3 nano-bricks, the greater and stronger the plasmon coupling-induced electromagnetic hotspots, endowing WO3-Au (2.1 wt%) with outstanding SERS sensitivity.
The plasmonic resonance of Au nanoparticles may be stimulated by visible light, which can boost the light-harvesting capacity of the WO3-Au hybrids. Furthermore, the activation process between Au and WO3 can accelerate carrier separation, improving photocatalytic activity and efficiency.
It was discovered in the study that the photocatalytic activity of WO3-Au (2.9 wt. percent) is lower than that of WO3-Au (2.1 wt. percent). Excess Au nanoparticles on WO3 may operate as recombination centers, slowing charge transfer and hence reducing photocatalytic efficacy.
Limitations
Although the study has carefully assessed the semiconductor hybrid, its results still have a limitation. These results are only applicable to semiconductors made up of a WO3-Au mixture. The values can’t be generalized for all semiconductors hybrid. Hence, for other semiconductors, new experiments and findings are essential.
In short, the research has thoroughly assessed almost all essential aspects of the specific type of semiconductor hybrids. The results would significantly increase their efficiency resulting in a massive boost to its lifetime, reduction in operational costs, and would result in a massive growth in its rapid commercialization.
References
Tang, L., Liang, S., Li, J. B., Zhang, D., Chen, W. B., Yang, Z. J., . . . Wang, Q. Q. (2020). Controlled Synthesis of Au Nanocrystals-Metal Selenide Hybrid Nanostructures toward Plasmon-Enhanced Photoelectrochemical Energy Conversion. Nanomaterials. https://www.mdpi.com/2079-4991/10/3/564
Volokh, M., & Mokari, T. (2020). Metal/semiconductor interfaces in nanoscale objects: synthesis, emerging properties and applications of hybrid nanostructures. Nanoscale Advances, 930-961. https://pubs.rsc.org/en/content/articlelanding/2020/na/c9na00729f
Zhang, J., Ji, M., Liu, J., & Xu, M. (2016). Metal/Semiconductor Hybrid Nanocrystals and Synergistic Photocatalysis Applications. Advanced Catalytic Materials - Photocatalysis and Other Current Trends. https://www.intechopen.com/chapters/49640
Zou, J. W., Li, Z. D., Kang, H. S., Zhao, W. Q., Liu, J. C., Chen, Y. L., . . . Ding, S. J. (2021). Strong Visible Light Absorption and Abundant Hotspots in Au-Decorated WO3 Nanobricks for Efficient SERS and Photocatalysis. ACS Omega, 28347-28355. https://pubs.acs.org/doi/10.1021/acsomega.1c04536
Disclaimer: The views expressed here are those of the author expressed in their private capacity and do not necessarily represent the views of AZoM.com Limited T/A AZoNetwork the owner and operator of this website. This disclaimer forms part of the Terms and conditions of use of this website.