Gas chromatography (GC) is a robust and widely used analytical technique for the separation and analysis of vapor-state chemical compounds. However, their bulky instrumentation and peak power requirements render them important for compact and field-appropriate versions of analytical instruments. This article delves into the concept of micro GC (μGC) and its important components.
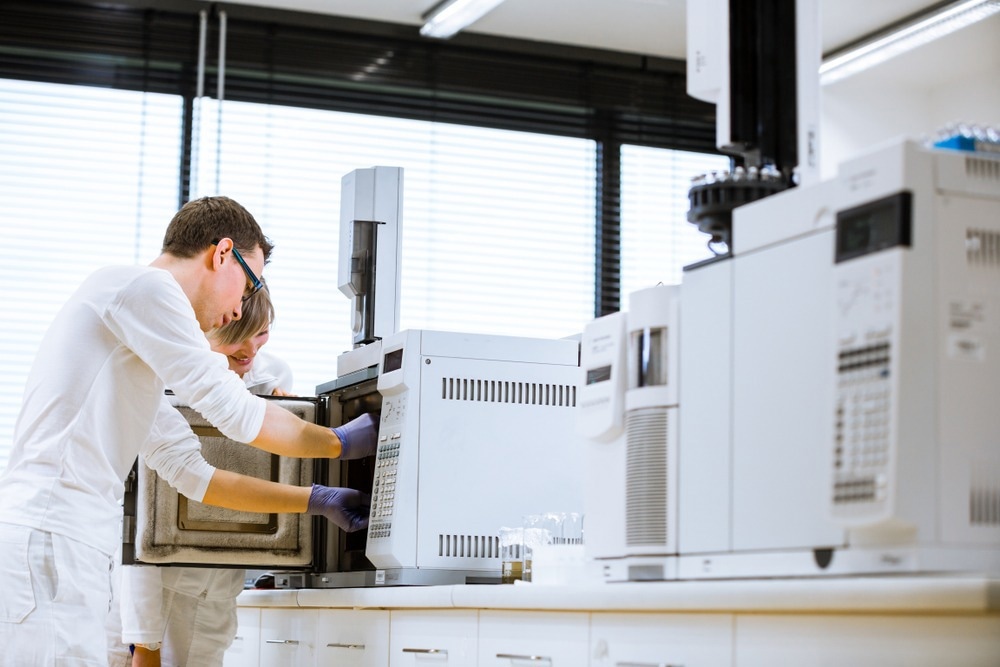
Image Credit: l i g h t p o e t/Shutterstock.com
How has Traditional Gas Chromatography Evolved?
Although the concept of chromatography was invented in 1900 by a Russian-Italian botanist, Mikhail Tsvet, the concept of Gas Chromatography (GC) surfaced only in the early 1950s by the University of Chicago’s team of researchers, led by Martin and James.
Early GC with limited capabilities could not analyze the separated components because of the lack of suitable detectors. However, the development of thermal conductivity, flame ionization, and other advanced detectors over time has made GC a robust chromatographic method.
GC has evolved over the years and has been diversified into various specialized techniques to analyze samples of any physical state. Thus, it has been adopted in various fields of research, including biochemistry, chemistry, forensic science, petrochemical analysis, etc.
Why Portable Gas Chromatography Devices?
There has been a recent surge in the demand for a quick analysis of complex mixtures at the site instead of a time-delayed analysis in the lab due to three main reasons:
- For quick decision-making from the results obtained.
- On-spot testing helps efficient sample collection.
- The composition of certain samples changes over time due to physical processes, including degradation, evaporation, and others. Hence, on-site analysis helps in analyzing the sample in its original state.
Over the past 40 years, the market has witnessed an increased supply of portable GC instruments designed in both commercial and research laboratories. The μGC helps in real-time analysis of gasses, providing accurate results in the field of forensics, healthcare and others.
The compact size, low power consumption, automatic operation, and cost-effectiveness make μGC highly reliable for on-site testing. The components of a μGC consist of a carrier gas source, pre-concentrator-injector, separation column, pump, detector, instrument control software, data acquisition, and analysis.
Working Principle of μGC
While the working principle of μGC is similar to that of traditional GC, its instrumentation has been downsized to a miniature version to enable greater portability and to meet other requirements.
When a mixture is allowed to pass through a μGC separation column, its components exit the column at different rates, resulting in a Gaussian-shaped distribution peak. Here, the peak width indicates the efficiency of the chromatographic process. That is, the narrower the peak, the greater the efficiency.
Based on the Van Deemter theory, proposed in the 1950s, a peak’s width may increase owing to factors including the mobile phase’s velocity and diffusion type in the column. This theory helped to adapt columns of different shapes and explain different equations.
Although an ideal peak should be symmetrical in shape, the peaks in real-world samples often have asymmetric shapes, affecting the separation performance, which is quantified as an asymmetry or tailing factor.
Another concept, the peak capacity, is used to measure the number of components that undergo complete separation by the column within a predetermined time frame. Furthermore, the limitation of μGC in separating very complex mixtures is overcome by two-dimensional (2D) chromatography, which uses two columns with different separation properties.
Columns Used in Micro Gas Chromatography
While the separation columns in conventional chromatography are circular, those in μGC are rectangular. Polyimide-clad fused silica capillary tubing is a commonly preferred capillary column in this type of chromatographic system because of its stationary phase film coating, which offers a high resolution.
Although narrower columns enhance the separation efficiency, they have a reduced sample capacity. This limitation is overcome by a multicapillary column (MCC), which facilitates parallel operations. Recently, semi-packed columns (SPCs) have gained significant attention. An array of micropillars inside the channels of the SPCs boosts the sample capacity by increasing the surface area.
Stationary Phase in μGC
Extensive research has been conducted to evaluate potent stationary phases in μGC, including graphitized carbon black (carbopack), polyethylene glycol (PEG)-coated graphitized carbon black (carbograph), porous polymer beads, and carbon molecular sieves.
Additionally, open and semi-packed columns, including polydimethylsiloxane (PDMS) and its derivatives, used to separate a mixture of benzene, xylene, and PEG (carbowax), were explored.
The most common coating methods used in chromatography are dynamic and static methods. Although the former method can provide a uniform stationary phase, there are chances of bubble formation. The latter method has a plug of stationary phase solution that is pushed through the column.
Although the dynamic coating method was faster than the static method, predicting the film thickness in the former coating method was challenging. Additionally, gold, metal-organic frameworks (MOFs), and room temperature ionic liquids (RTILs)-based stationary phase are the new classes that have garnered attention.
Microfabricated Preconcentrator
Preconcentration helps purify and concentrate the samples before introducing them into μGC. While the conventional preconcentrator has stainless steel or glass tubes filled with adsorbent, the microfabricated preconcentrator uses a micro hot plate coated with a surfactant-templated sol-gel adsorbent, facilitating the quick desorption of analytes.
Detectors
The stream of gas from the column is passed through a detector to obtain a signal that is in turn used for qualitative or quantitative analysis of components in a mixture. Various detectors with different sensitivities, strengths, and areas of application are used in μGC.
The detectors commonly used in μGC include surface acoustic wave (SAW) devices, thermal conductivity detectors (TCD), chemi-capacitive sensor arrays, chemiresistor arrays, photoionization detectors (PID), nanocantilever, electron capture detectors (ECD), and flame ionization detectors (FID).
Conclusion
Overall, interest in μGC for on-site analysis of samples has surged in the recent past. Consequently, this chromatographic technique has been significantly transformed over time in different aspects to enhance its performance. However, most μGC studies have been limited to simple hydrocarbons.
More from AZoM: An Overview of Flue Gas Analysis
References and Further Reading
Biswas, P., Zhang, C., Chen, Y., Liu, Z., Vaziri, S., Zhou, W., & Sun, Y. (2021). A portable micro-gas chromatography with integrated photonic crystal slab sensors on chip. Biosensors, 11(9), 326. https://www.mdpi.com/2079-6374/11/9/326
Who and When was Gas Chromatography invented? (Accessed on 13 August 2023)
Regmi, B. P., & Agah, M. (2018). Micro gas chromatography: an overview of critical components and their integration. Analytical chemistry, 90 (22), 13133-13150. https://doi.org/10.1021/acs.analchem.8b01461
Disclaimer: The views expressed here are those of the author expressed in their private capacity and do not necessarily represent the views of AZoM.com Limited T/A AZoNetwork the owner and operator of this website. This disclaimer forms part of the Terms and conditions of use of this website.