By Surbhi JainReviewed by Susha Cheriyedath, M.Sc.Jun 24 2022
In a review recently published in the journal Bioprinting, researchers presented a comprehensive review of the development of cardiac tissue using cardiovascular 3D bioprinting.
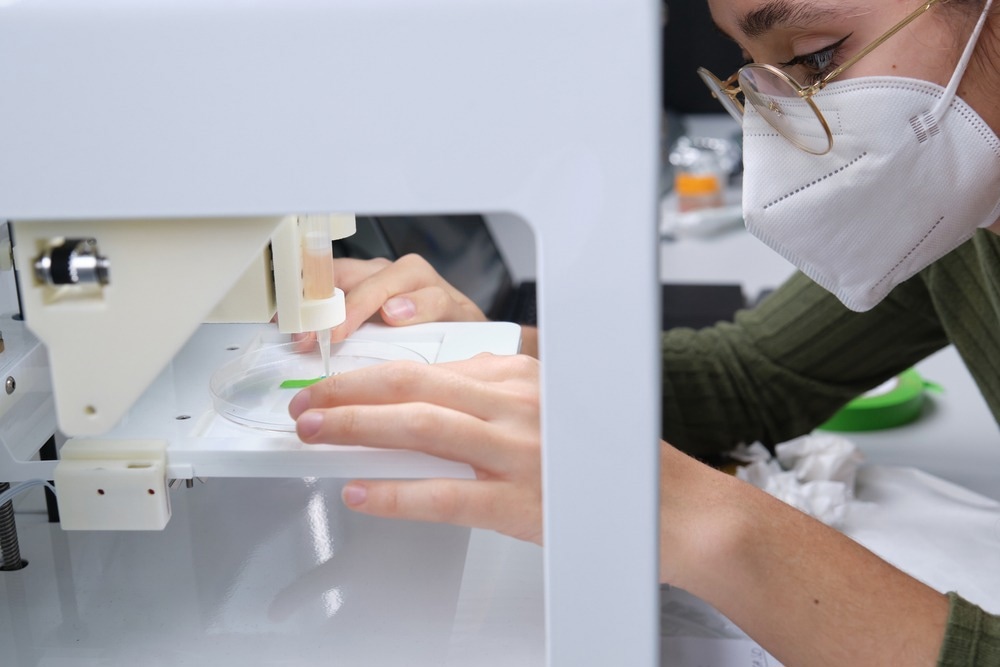
Study: Cardiovascular 3D bioprinting: A review on cardiac tissue development. Image Credit: Ladanifer/Shutterstock.com
Background
One-third of all deaths worldwide are caused by cardiovascular diseases (CVDs). The major cause of CVDs, which are characterized by severe inflammation and cardiomyocyte death caused by hypoxia, is thought to be myocardial infarction (MI). The main clinically available treatments for end-stage CVDs are prosthetic devices, coronary artery bypass grafts (CABGs), and heart transplantation; however, cardiac transplantation treatments are clinically limited due to organ shortages and immunogenic rejection following transplantation.
The low local cell retainability caused by blood flow and immunogenic inconsistency, which leads to low integration into the host tissue, have persisted as major hurdles despite several attempts over the past ten years to enhance cell transplantation techniques.
The native tissue qualities can be replaced by engineering a transplantable three-dimensional (3D) microenvironment for enhanced cell-cell and cell-matrix interaction. To increase the effectiveness of cardiac tissue engineering techniques, novel bioengineering techniques are being researched. Among these cutting-edge techniques, 3D bioprinting, a formidable bio-fabrication technology derived from additive manufacturing, holds a special promise for the creation of intricate 3D structures. In the past ten years, a variety of bioprinting methods based on extrusion, inkjet, and laser technologies have been developed.
About the Study
In this study, the authors presented a thorough overview of the methods used to create cardiovascular functional tissues, which ranged from the development of bioinks made of cells, hydrogels, and biofactors to bioprinting procedures and pertinent biophysical stimulations that were responsible for tissue maturation and functions at the level of the tissue. This review also emphasized investigations into 3D bioprinting of heart tissue and further discussion of its issues and prospects.
The team illustrated various cell sources and hydrogels that were employed in the creation of bioinks. Various architectural layouts, stimulation strategies, and bioprinting approaches that improve the functionality of biological constructions were examined. The researchers presented a discussion of cardiovascular bioprinting issues and viewpoints based on research from the previous 10 years.
Observations
The printed passivation bioink was evaluated for resistance change due to deterioration and mechanical evaluation, and both revealed no appreciable resistance change and 135% elongation. The biological construct was supported by gelatin baths, which could be un-crosslinked at a temperature of 37 °C.
The use of morphologically homogeneous microparticles with a diameter of 20 µm, which offered support with improved mechanical qualities, was another enhancement of FRESH v2.0. In contrast to the 7% of uncultured cells that were oriented in the same degree range, 40% of hMSCs grown on microchannels had their orientation set to 0 to 10 degrees. Compared to samples with 1000 µm spacings, stem cells cultivated on microchannels with a 500 µm spacing were more stretched in the direction of the gelatin pattern.
Improved cell-cell integration, well-organized cell alignment, vasculature incorporation, better management of complicated designs, and better functionalities were a few examples of the substantial advancements in cardiovascular bioprinting. The introduction of more biologically sensitive bioinks and the development of bioprinting techniques were largely responsible for them. The latter was made possible by the use of regenerative cell technology and more varied natural and synthetic hydrogels. Although significant progress was made, some obstacles prevented the manufacturing of functional large-scale cardiac tissue. The limitation of the tissue-specific activities caused by incomplete cardiomyocyte maturation continued to be a major obstacle, which made it even more difficult to integrate the host tissue.
Efforts to use physiologically relevant stimulations to induce tissue-specific gene expression were proven impressive. Reaching a suitable population of fully developed cardiomyocytes, however, was still a significant issue. Another issue that greatly limited the clinical use of bioprinted cardiac tissues was the absence of dense vasculature in the construct. In order to distribute heterogeneous cell types uniformly throughout the scaffold, improve cell survival, and enhance cellular activity, efficient mass transfer inside the construct was a crucial challenge. The provision of a vascular network with a density of about 3000 cells/mm2 demanded novel bioprinting techniques with better resolutions and improved modeling properties.
The construct's mechanical properties could have a significant impact on how well it integrated with the host system and how cells migrated toward cardiomyocytes. The arrangement of cardiomyocytes in cardiac patches was influenced by the bioprinting of bioinks with various architectural configurations, which increased proliferation and biological processes including notch signaling.
Bioprinting with bioinks made of hydrogels with high conductivity, such as CNTs, was an additional method for the promotion of cardiomyocytes' increased cell proliferation and improvement of the functional gene expression that resulted in contractility. Cardiomyocytes, which formed their own ECM identical to actual tissue in the composition structure after the in vivo implantation, were used to create cardiac patches using new techniques including bioprinting scaffold-free cells. One method that emphasized endothelial cells' influence on cardiomyocyte survivability and function was bioprinting layers of the endothelial cells between layers of cardiomyocytes.
Conclusions
In conclusion, this study elucidated that the techniques, such as growing cardiomyocytes after creating endothelialized constructions, were used to imitate the structure of heart tissue. It was demonstrated that cardiomyocytes with anisotropic endothelialized structures were more orientated and contract at higher frequencies.
The main methods used to enhance cardiac tissue functions included the co-culture of cardiomyocytes and endothelial cells, the combination of hydrogels with high conductivity additives, and the architecture of 3D bioprinted constructs. These methods affected cell proliferation, aggregation, and differentiation to produce tissue-like contractile structures with beating areas.
The authors believe that the fabrication of bioprinted tissues in zero- or microgravity can promise further capabilities in the field of cardiovascular bioprinting, which is an interesting new angle. They mentioned that the microgravity conditions recommend using lower viscosity bioinks and more acceptable resolutions when printing at the single-cell size, in contrast to the current scenario on Earth.
More from AZoM: Building Durable and Sustainable Futures with Graphene@Manchester
Disclaimer: The views expressed here are those of the author expressed in their private capacity and do not necessarily represent the views of AZoM.com Limited T/A AZoNetwork the owner and operator of this website. This disclaimer forms part of the Terms and conditions of use of this website.
Source:
Kalhori, D., Zakeri, N., Zafar-Jafarzadeh, M., et al. Cardiovascular 3D bioprinting: A review on cardiac tissue development. Bioprinting e00221 (2022). https://www.sciencedirect.com/science/article/pii/S2405886622000318