Sponsored by AdmesyJul 12 2018
The science of the measurement of light in terms of how the human eye perceives its brightness is known as photometry, which reveals differing sensitivity to different wavelengths of visible light.
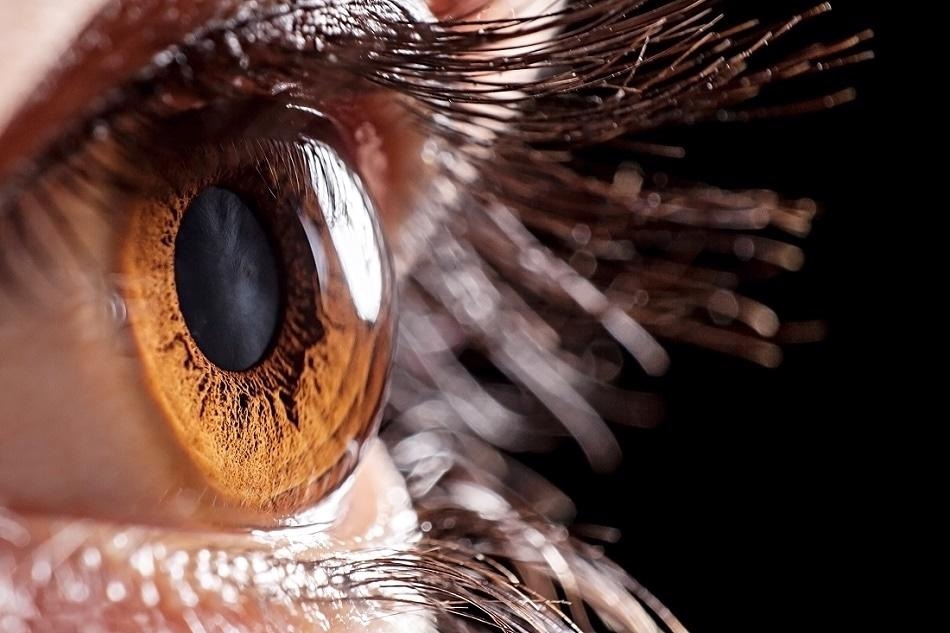
Image Credit: air009/shutterstock.com
Photometric measurements bias the radiant power at each wavelength with a luminosity function, which models human spectral sensitivity, to account for this variation. The photopic sensitivity function is typically this weighting function.
Photometric measurements are crucial in the quality control and development of any lighting that is to be utilized in a human environment. This covers everything from displays such as those found in automobile dashboards and televisions, to the LEDs and generic lighting used in buildings.
Defining the Average Observer
Photometry, as outlined above, is the calculation of visible light based on the reaction of a typical human eye. Physical, physiological, and psychological characteristics all affect the eye’s response and these factors vary greatly from person to person. In addition to this, the human eye has two types of cells which are functional for low light levels (rods) and high light levels (cones). This means an average observer is difficult to define.
In 1924 a series of experiments were conducted by The Commission Internationale de l’Eclairage (CIE) to measure the response of the human eye to visible light. They found that there are two vision response curves that dictate photometric measurements.
- The photopic function for high light conditions was given the spectral luminous efficiency function V(λ) to characterize the daylight vision of an average human observer.
- At low light levels, the CIE defined the scotopic function V’(λ) to characterize the dark-adapted eye response.
The Limits of Human Visual Perception
Although the visible spectral range is typically defined as 380 to 780 nm, the eye can perceive colors in the range of wavelengths from roughly 380 to 700 nm. The wavelength of the light determines the color that is perceived and the amount of light energy detected by the eye at a specific wavelength determines the apparent intensity of the color.
The eye’s response is highest in the region around 555 nm wavelength. The majority of individuals are unable to perceive blue below 400 nm or red above 700 nm and as a result, the photopic response is virtually zero in these regions. The scotopic response peak tends towards the blue region. This is because in the dark-adapted eye, the cone cells (color receptors) shut down and this leaves monochromatic rod cells as the primary sensors with a maximum sensitivity around 507 nm.
The Laws and Types of Photometric Measurement
There are two principal laws utilized to produce photometric measurements. They are known as the inverse square law and the cosine law.
The inverse square law determines the correlation between illumination from a constant-intensity source and its distance from the surface. The inverse square law states that the intensity per unit-area on the surface varies in inverse proportion to the square of the distance between the source and surface. This means that consecutive illuminance measurements are only as precise as the control of surface to source range.
Lambert’s cosine law states that the strength of light on a surface of a fixed area varies with incident angle. When light hits a surface obliquely, the illumination of the surface is directly proportional to the cosine of the angle θ between the direction of the incident light and the surface normal. A surface obeying the law has the same radiance when viewed from any angle and exhibits Lambertian reflectance. To the human eye, the brightness looks the same.
When measuring displays, a number of different photometric measurements can be obtained, these include:
- Luminous Intensity: It is defined as the quantity of luminous flux emitted uniformly into a solid angle. This assesses the source’s divergence and direction. This measurement is not applicable to collimated light sources and is inaccurate for non-uniform emitters. The rudimentary unit of luminous intensity is the candela and is equal to one lumen per steradian. The detector’s area (or the area prescribed by the aperture in front of it), and its distance from the light source must be known in order to calculate luminous intensity. With those, the solid angle can be calculated and then divided into the flux reading.
- Luminous flux: The central quantity used in photometry is luminous flux and this is described as the visible light energy per second radiated by a source. Luminous flux is conveyed in lumens. It is a measure of a visible light sources whole optical output in all directions. Recording this measurement requires focusing a source’s full power on a detector. This can be accomplished by utilizing an integrating sphere in 4π configuration or by using a gonio system.
- Luminous Energy: Luminous energy is defined as the rate of flow of flux and is expressed in lumen-seconds. Normally, it is applied to pulsed or flashed sources.
- Luminance: Luminance or photometric brightness is defined as the flux from a relatively flat and uniform surface, which may be reflected or emitted. Luminance has the units of candelas per square meter. When measuring luminance, the detector field-of-view must be restricted, and its angle calculated.
- Illuminance: Illuminance describes the amount of visible light incident upon a set surface area. The unit, lumen per square meter, is also known as lux. Illuminance measurements are prone to errors introduced by off-axis light which must be cosine corrected.
Key Applications of Photometric Measurements
Luminance meters and imaging photometers are used for the accurate uniformity and intensity testing of displays in the following;
- Automotive dashboards
- Mobile phones
- Instrument panels
- Night-vision devices
- Computers
- Tablets
- TV’s
The luminance of the small characters on instrument panels and cockpit displays is highly controlled in the aerospace and automotive industries. Applications such as these utilize computerized quality control with 2D imaging systems or spot photometers.
In other applications, photometers are used to measure the contrast ratios of OLED flat panel displays, cathode-ray tubes and liquid crystal displays. Some typical photometric applications include the optical density or transmittance of filters, illuminance of theatre screens, ISO ergonomic testing of computer monitors, and the uniformity of projection systems.
Achieving Ideal Photometric Measurements
The main focus of photometry is to recreate the spectral response of the human eye. Photometer sensors must be spectrally corrected, however, as electronic sensors have very distinct response characteristics that are completely different from the defined CIE standard observer. This can be done in two different ways: optimizing via software or hardware.
For hardware, the integration of the spectral response of the filter and photodiode is optimized. Filter-matching involves layering colored-glass filters over an optical detector. Each element attenuates at selective wavelengths until the detector’s response replicates the CIE curve.
Accurately matching the response is dependent on the reproduction and quality of the components and may be checked for every combination, which is a downside to this technology. An Advantage, however, is the ability to apply this sensor type to high speed measurements, which also allows for measuring flicker, response times and start-up behavior. Most common photometers apply this type of technology.
Photometers operate by absorbing light through input optics that have been specially designed, passing it through a spectral modifying filter and then measuring the light that passes through with a photosensitive detector. The filter is carefully trimmed to adjust the detector response in such a way so that it matches the CIE photopic or scotopic function.
The detector converts the incoming light energy into an electrical signal, which is then amplified and displayed. The electrical signal that is measured is an objective measurement of light as perceived by a human observer. This is because the filter/detector combination approximates the eye response.
There are a number of factors the performance of a photometer used for a particular application depends on. For example, acceptance angle of the optics, the closeness of the photopic filter's match to the CIE V (λ) function, the stability and linearity of the detector and quality of the photometric calibration.
The repeatability and accuracy of results rely upon on the spectrum of the source being measured. This is because the photopic filter in most photometers does not seamlessly match the CIE V (λ) function correctly. This incorrectness is expressed as its f1’ value.
LEDs, cathode-ray tube phosphors, and discharge lamps are highly saturated sources, so can pose issues because their narrowband spectra differ largely from the broadband incandescent sources used to calibrate most photometers. Errors caused by lens flare and polarization and can severely affect the measurement of liquid crystal displays.
Spectrometers from Admesy for Photometric Measurements
Software processing over spectral measurements is another technology utilized to capture photometric data. The exact spectral power distribution of the light source can be determined accurately when applying a calibrated spectrophotometer to it.
Using software processing, the V (λ) is applied, removing any influences like the f1’ values. Photometric data can be determined with even more precision, especially when switching between emitting samples with different spectral power distributions. Besides the Y value, the same high accurate determination by a spectrometer also holds for the CIE 1931 X and Z functions. This is the exact reason that for each display type with a unique spectral power distribution, colorimeters have to be calibrated using a spectrometer.
Rhea series is one of Admesy’s spectrophotometers. It is a flexible spectrometer platform with configurable spectral measurement for the range between 200 and 1100 nm. Versions covering the VIS range have integrated software processing for direct readout of highly accurate CIE functions.
The instruments sensors, slit size and gratings can be chosen when ordering for custom spectral measurements. Together with a high-end cooled CCD sensor, the Rhea has the flexibility of a spectrometer for OEM integration, lighting, displays and appearance measurements in both laboratory and production settings.
The Hera is an extremely concise, compact spectrometer with excellent dark and linearity compensation. Similar to the Rhea, applications for the device can range from 24/7 inline use for production environments to development and sample-based quality inspection.
All instruments are available with a fixed (lens or cosine corrector) or fibre optics setup. The instrument is configured to make a range of measurements which include spectral analysis, color in Yxy, and parameters (for example, color rendering index (CRI), correlated color temperature (CCT) and PAR).
These are flexible instruments ideal for a range of display, lighting and analysis applications which match the photometry requirements of the CIE functions.
References & Further Reading
- Stimson, A. Photometry and Radiometry for Engineers. Wiley-Interscience (1974).
- CIE 41 — Light as a True Visual Quantity: Principles of Measurement. CIE, Vienna (1978).
- Whitehead, Lorne A.; Mossman, Michele A. (2006). "Jack O'Lanterns and integrating spheres: Halloween Physics". American Journal of Physics. 74 (6): 537–541.
- Miller, K. How to Make Accurate Light Measurements. Photo Research, Chatsworth, Calif. (1985).
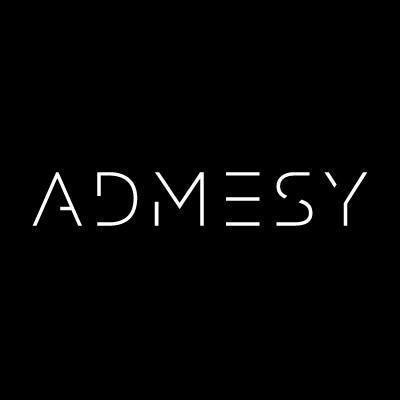
This information has been sourced, reviewed and adapted from materials provided by Admesy.
For more information on this source, please visit Admesy.